|
Mechanism of Boundary Film Formation from n-Hexadecane
M. Makowska Institute for Terotechnology, Radom, Poland
C. Kajdas Warsaw University of Technology, Plock,Poland, and
M. Gradkowski Institute for Terotechnology, Radom, Poland
Published in: Lubrication Science 16-2, February 2004. (16) 101
Abstract
Keywords: n-hexadecane, surface film, boundary lubrication, film formation mechanism, FTIR microspectrophotometry, XPS
It is widely acknowledged that the process of surface film formation by tribological additives is very complex, because even saturated hydrocarbons - as major components of lubricating oils - undergo chemical changes under boundary lubrication conditions. To gain a better understanding of the tribochemical changes of paraffinic hydrocarbons, research was carried out using n-hexadecane, which is widely used as a low-viscosity model base oil. It has been hypothesised that the interactions between the products of n-hexadecane triboreactions and steel surfaces are mostly initiated by the mechanical action of the system. Wear tests were performed, using a pin-on-disc machine. Fourier transform infrared microspectrophotometry and X-ray photoelectron spectroscopy were used to analyse the wear tracks on the steel discs. Based on the analytical data obtained, a model of boundary film formation from aliphatic hydrocarbons is proposed.
BACKGROUND
It is widely acknowledged that the process of boundary film formation by tribological additives is very complex, because even hydrocarbons, which are major components of lubricating oils, undergo chemical changes under boundary lubrication conditions [1]. Therefore, to understand the triboreactions of aliphatic hydrocarbons better, it is necessary to carry out research using the simplest compounds, for example n-hexadecane. This is a non-polar compound and thus does not
Table 1 Tribological test conditions |
Ball material | 52100 steel |
Ball diameter (mm) | 6.35 |
Disc material | 52100 steel |
Disc diameter (mm) | 25.4 |
Load (N) | 9.81 |
Sliding speed (m/s) | 0.25 |
Sliding distance (m) | 500 |
Temperature and humidity | Ambient
|
Table 2 XPS analysis conditions |
Radiation source | Mg Ka, 250 W |
Reference line | C 1s.284.6eV |
Energy resolution (eV) | ±0.2 |
Test region diameter (mm) | 0.8 |
Pass energy (eV) | 29.35 |
Analysis time (min) | 1.07
|
|
compete with tribological additives in surface adsorption processes. Therefore it is widely used as a low-viscosity model base oil and reference fluid in testing new compounds as potential candidates for tribological additives. Accordingly, the present work aims to investigate this specific tribochemical issue without the influence ofantiwear/extreme-pressure additives using only pure n-hexadecane as a lubricant.
Early work [2] demonstrated that the sliding behaviour of steel lubricated by n-hexadecane under boundary lubrication conditions could be related to chemical reactions at the sliding surfaces involving metal, hydrocarbon, and oxygen. From the literature, it is known that oxidation processes under friction conditions are very specific. Some processes occur simultaneously and are controlled by different mechanisms. Major tribo-oxidation compounds from n-hexadecane are related to
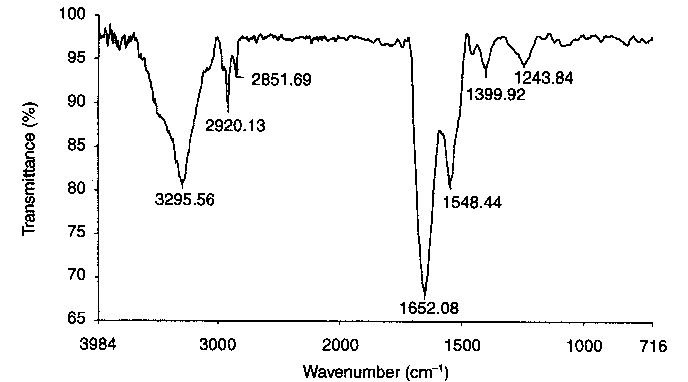 |
Figure 1 FTIR spectrum of steel disc surface after n-hexadecane-lubricated friction test (after n-hexane washing) [4]
|
oxygenates other than carboxylic acids. This suggests that the reactions are initiated by mechanical action of the tribosystem and occur at sites where fresh metal surfaces are exposed by rubbing.
This work presents results concerning the behaviour of n-hexadecane in a tribosystem at ambient temperature. It is hypothesised that interactions between the products of n-hexadecane triboreactions and steel surfaces are mostly initiated by the mechanical action of the system.
EXPERIMENTAL
Rubbing tests were performed using a T-11 ball-on-disc machine, produced at the Institute for Terotechnology, Radom, Poland. All experiments were carried out under boundary lubrication conditions (see Table 1) established on the basis of ASTM G 99-90 and previous experiences [3]. The elements of the friction pairs were washed in n-hexane and stored in a dry box (above silica gel) before and after each run. n-Hexadecane (>99%, Merck) was used as a lubricant without any additional purification.
To analyse (ex situ) organic products layered in the wear tracks on the steel discs, Fourier transform infrared microspectrophotometry (FTIR) in the wavenumber range
Table 3 Interpretation of spectral frequencies in Figure 1 |
Frequency (cm -1) | Nature of vibration | Reference
~3300 | 3600 - 3200 | Bonded —O—H stretch | 5-8 |
~3300 | ≡C-H stretch | 9 |
3500 - 3000 | —NH stretch | 9, 10 |
~1650 | 1630 - 1600 | —O—H bend (in water) | 6, 9, 11, 12 |
1680 - 1620 | —C═O stretch | 9, 13, 14 |
1680 - 1620 | —C═C stretch | 9, 15 |
1654 | —C═N-stretch | 15 |
1650 - 1620 | —N—H bend | 10 |
~1550 | ~1534 | —C═O stretch | 16 |
1580 - 1560 | —COO Stretch (in carboxylates) | 5, 6, 12, 17 |
1530 - 1520 | —C═O, —C═C stretch (in complex compounds) | 12 |
1570 - 1510 | —N—H bend | 10
| |
4000-700 cmSup>-1 (resolution 4 cm-1, 100 scans) was used. All spectra were corrected by excluding the carbon dioxide band, smoothing, and normalising of the baseline. This software processing of the spectra showed no influence on their appearance.
X-ray photoelectron spectroscopy (XPS) was used to determine the types of chemical bonds present in the compounds. The C 1s, O 1s and Fe 2p spectra were recorded under the conditions listed in Table 2.
RESULTS
FTIR analysis of organic compounds present on the steel discs after the friction tests was the starting point for further detailed investigations. A basic advantage of this method is the possibility of obtaining spectra immediately from the friction pair surfaces. Figure 1 shows the FTIR spectrum recorded on the steel disc wear scar lubricated during rubbing with n-hexadecane. The interpretation of the characteristic spectral bands
Table 4 Elemental composition of products layered on the steel surface during friction |
Element | Binding energy (eV) | Concentration (at. %)
C 1s | -288.5; -285 | 60.74 |
O 1s | -532 | 30.89 |
Fe 2p | -711 | 8.37
| |
around 3300, 1650, and 1550 cm-1 appearing in the spectrum is given in Table 3. This frequency range is emphasised because of its useful diagnostic value. In the region mentioned, there are overlapping bands that correspond to different organic structures. This is one reason for the various assignments in the literature and the difficulty of adequate interpretation.
Comparison of the spectrum shown in Figure 1 with an n-hexadecane spectrum taken from the same disc (presented in a previous work [18]) led the authors to suppose that tribochemical reactions occur on the steel surface under dynamic rubbing test conditions. The results indicate that there were carboxylic acid salts, unsaturated compounds, complex compounds, and/or nitrogen-containing compounds on the steel surface. However, EDS analysis excluded the participation of nitrogen in the chemical structures of the products [19]. Further investigations are intended to identify the products' chemical structure.
On the basis of the binding energies determined by means of XPS (see Table 4) the types of chemical bond in the product molecules were investigated. The atomic percentage of carbon (about 60%) confirms the presence of an organic layer on the steel rubbing surface.
The C 1s spectrum contains two signals: ~288.5 eV, assigned to the C═O bond, and ~285 eV, assigned to the C—O bond in carboxylates [20,21]. The 711 eV peak dominating in the Fe 2p region corresponds to a compound form of iron (elemental iron binding energy is 706.4 eV [22]). This is typical of the Fe—O bond, in such compounds as, for example, oxides, hydroperoxides, and carboxylates [20]. In the O 1s spectrum a multiple peak has been identified at ~532 eV. Figure 2 (overleaf) shows the result of its separation. The low binding energy of 529.81 eV is the characteristic location for oxide oxygen, but also correponds to oxygen in organic compounds. The location of the
531.61 eV peak which dominates in this spectrum corresponds to the presence of organic compounds, and it can be related to carboxylates [20] or to complex compounds of iron.
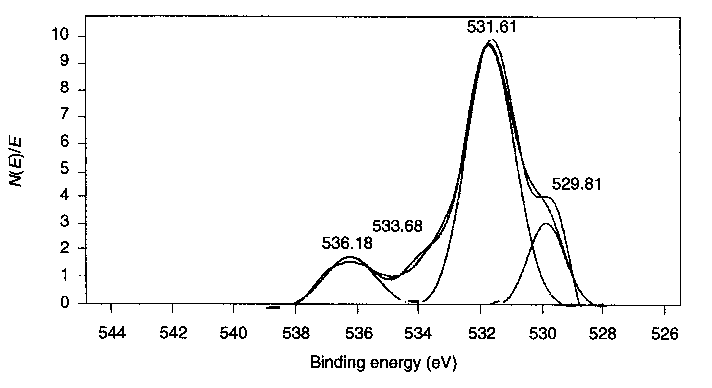 |
Figure 2 Result of separation of overlapping peaks in O 1s XPS spectrum
|
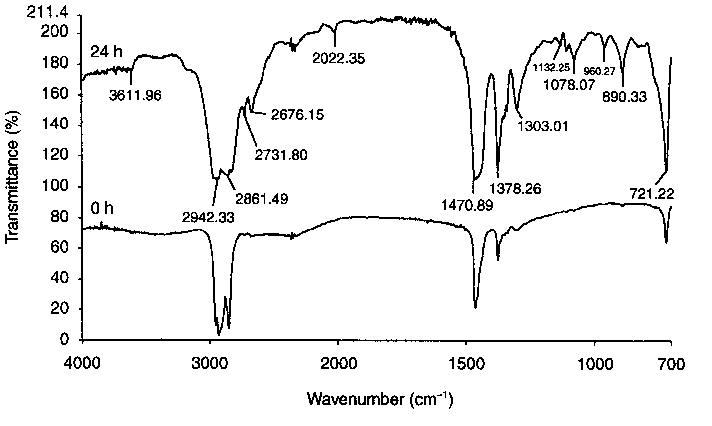 |
Figure 3 Comparison of FTIR spectra of n-hexadecane taken at the start of the static test (lower trace) and of products forming from n-hexadecane after 24 h (upper trace)
|
Table 5 Interpretation of spectral frequencies in Figure 3 [9, 10] |
Frequency (cm -1) | Nature of vibration
2942 | —CH3 stretch |
2861 | —CH2— stretch |
2732 | —CH stretch in —CHO |
2676 | Bonded —O—H stretch |
1471 | Asymmetric —CH3 bend |
1378 | Symmetric —CH3 bend | |
|
Frequency (cm -1) | Nature of vibration
1303 | —CH2— oscillation |
1132 | —CH3 oscillation |
1078 | —C—OH stretch |
960 | —C—H deformation in —C═C—H |
890 | —C—H deformation in —C═C—H |
721 | —CH2— oscillation in an at least five-carbon chain | |
|
The binding energy of 533.68 eV corresponds to the C-O bond in carboxylate structures [23]. The signal at 536.18 eV offers no significant information because it is located beyond the interpretative oxygen region.
A test was performed to ascertain whether the same changes would take place under static oxidation conditions (i.e., no friction testing). A drop of n-hexadecane was put on several steel discs and oxidised at ambient temperature (this temperature was chosen to match the friction test temperature) in air for about 144 h. At regular intervals (every 24 h) the disc surface was investigated by FTIR, without solvent washing. Figure 3 (upper trace) shows an example of a spectrum after 24 h of the static test and Table 5 summarises possible assignments of the recorded spectral frequencies.
In all tests, regardless of the time elapsed since the n-hexadecane was put on the steel, the FTIR spectra were the same shape as the spectrum recorded for the rubbing surface without n-hexane washing. Furthermore the spectra obtained were different from the initial re-hexadecane spectrum (Figure 3, lower trace). It can thus be assumed that during static tests some oxidation reactions took place. These led to the generation of similar compounds in the bulk lubricant during both static and dynamic tests, but with additional products generated on the steel surface during rubbing tests.
In order to determine the degree to which oxidation products were bonded to the surface, after the static tests the discs were washed in re-hexane and again investigated using FTIR. No organic compounds were found on the steel surface. This means that only under friction conditions are organic products so strongly bonded to the steel surface that they are not washed off by the non-polar solvent.
SURFACE REACTION MECHANISM
A model of the process of boundary film formation from aliphatic hydrocarbons (n-hexadecane) is proposed based on earlier experimental results [24] and their specific interpretation [25]. It seems most probable that re-hexadecane in the first stages oxidises to alcohols, carboxylic acids, and other oxygenates. This was shown from gas chromatography/mass spectrometry analysis of bulk re-hexadecane from a tribological test chamber, after friction [19, 26]. Furthermore the similarity of FTIR spectra taken from n-hexadecane-lubricated wear tracks to those from wear tracks lubricated with alcohols clearly shows that, during friction, n-hexadecane oxidises to alcohols.
The reaction of low-energy electrons emitted during friction [24, 27] with lower-molecular-weight paraffins, for example n-hexane, causes the formation of reactive intermediates, i.e., negative ions and free radicals:
CnH2n+2 + e¯ → [CnH2n+1]¯ + H•
In this way the lubricated contact region becomes a specific chemical reactor in which free-radical reactions and reactions of anions with positively charged rubbing surfaces proceed simultaneously, enhanced by high temperature. To obtain negative ions of higher-molecular-weight paraffins, for example, n-hexadecane, HO¯ ions have to be attached [28]:
CnH2n+2 + OH¯ → [CnH2n+1]HO¯ (m/e = M + 17)
Previous work [24] clearly demonstrated that the stability of such (M + HO¯) ions decreases with a decrease in the molecular weight of the n-paraffin; the intensity of the M + 17 peaks increases from n-heptane to n-nonane in the ratio 1:2.5:6. The dehydrogenation process of M+17 ions leads to the formation of alkoxide ions [25]:
CnH2n+2OH¯ - H2 → [CnH2n+1]O¯
which can also react with the friction surface, forming Fe-O bonds, and/or oxidise to form reactive carboxylate species.
CONCLUSIONS
Using a ball-on-disc tribometer under steel-on-steel boundary lubrication conditions, a study was made of the tribochemical changes of ra-hexadecane initiated by friction. In the initial stages of the process, some multidirectional chemical reactions cause fragmentation of the hydrocarbon chain. The polar compounds (e.g., alcohols, carboxylic acids) formed in this way can interact with the steel surface. The interactions occur between functional groups and iron, iron oxide, or iron hydroperoxide. Some of these compounds are bonded to the steel surface and are not washed off by a non-polar solvent. Application of the FTIR and XPS techniques to the analysis of the surface films and deposits in the contact region gave evidence of the formation of compounds with Fe-0 bonding (salts and/or chelates).
References
- Hsu, S.M., et al., "Tribochemistry induced by nano-mechanical scratches", Proc. Int. Tribology Conf., Nagasaki, 2000.
- Fein, R.S., and Kreuz, K.L, "Chemistry of boundary lubrication of steel by hydrocarbons",
- ASLE Trans., 8 (1965) 29-38.
- Kajdas, C., et al., "Investigation of tribochemical behaviour of some unsaturated organic additives in steel-steel contact", Proc. Symp. on Lubricating Materials and Tribochemistry, Lanzhou, 1998.
- Kajdas, C., Makowska, M., and Gradkowski, M., "Influence of temperature on tribochemical reactions of n-hexadecane", Lubrication Science, 15, 4 (2003) 329-40.
- Hu, Z.S., et al., "Tribochemical and thermochemical reactions of stearic acid on copper surfaces studied by infrared microspectroscopy", Trib. Trans., 35, 1 (1992)189-93.
- Gore, R.C., et al., "Infrared absorption of aqueous solutions of organic acids and their salts", Anal. Chem., 21, 3 (1949) 382-6.
- Powell, J.R., and Compton, D.A.C., "Automated FTIR spectrometry for monitoring hydrocarbon-based engine oils", Lubr. Eng., 3 (1993) 233-9.
- Cann, P.M., and Spikes, H.A., "Fourier-transform infrared study of the behavior of grease in lubricated contacts", Lubr. Eng., 48, 4 (1992) 335-43.
- Smith, A.L, Applied Infrared Spectroscopy, John Wiley & Sons, New York, 1979.
- Silverstein, P.M., et al., Spectrometric Identification of Organic Compounds, John Wiley & Sons, New York, 1981.
- Voort, F.R., et al., "Monitoring the oxidation of edible oils by Fourier transform infrared Spectroscopy", J. Am. Oil Chem. Soc., 71, 3 (1994) 243-53.
- Nakamoto, K., Infrared and Raman Spectra of Inorganic and Coordination Compounds, John Wiley & Sons, New York, 1989.
- Reich, R.A., and Mendoza, E., "Can fatty esters exhibit extreme pressure behavior when used as boundary additives in hot rolling aluminum metal?", Lubr. Ing., 8 (1998) 10-16.
- Morales, W., et al., "A new antiwear additive/surface pretreatment for PFPE liquid lubricants", Trib. Trans., 40, 2 (1997) 321-9.
- Zielinski, W., and Rajca, A. (eds.), Spectroscopic Methods and Their Application to Identification of Organic Compounds, WNT, Warsaw, 1995 (in Polish).
- Zhang, J., et al., "The friction and wear behaviors of some O-containing organic compounds as additives in liquid paraffin", Wear, 219 (1998) 124-7.
- Hurley, S., et al., "Lubrication and reflow properties of thermally aged greases", Trib. Trans., 43, 1 (2000) 9-14.
- Kajdas, C., et al., "Tribochemistry of hexadecane under boundary lubrication conditions", Proc. 9th Nordic Symp. on Tribology, Porvoo, 2000.
- Makowska, M., "Investigation of tribochemical changes of aliphatic hydrocarbons under friction conditions", PhD thesis, Lodz, 2001 (in Polish).
- Liu, W., etal., "Dispersion of nano-particles in mineral oil - a super lubricant", Proc. Symp. on Lubricating Materials and Tribochemistry, Lanzhou, 1998.
- Ng, L.M., et al., "Surface chemistry of perfluoro ethers: an infrared study of the thermal decomposition of (C2F5O on Al2O3, Langmuir, 11 (1995) 127-35.
- Cavdar, B., and Ludema, K.C., "Dynamics of dual film formation in boundary lubrication of steels". Wear, 148 (1991) 329-46.
- Zhang, S., and Liu, Q., "Mechanisms of wear of metal by nitrile rubber under boundary lubrication conditions", Proc. Int. Conf. on Tribology, Beijing, 1998.
- Kajdas, C., "Negative ionisation of n-paraffins C6-C9, C20, C22 and C24 in EA-mass spectrograph", Rocznik Chemiczny, 45 (1971) 1771-5 (in Polish).
- Kajdas, C., "Tribochemistry", Proc. World Tribology Congress, Vienna, 2001.
- Makowska, M., et al., "Tribochemical reactions of aliphatic hydrocarbons on steel surface", Tribologia, 3 (2001) 331-40 (in Polish).
- Kajdas, C., "About a negative-ion concept of the antiwear and antiseizure action of hydrocarbons during friction", Wear, 101 (1985) 1-12.
- Ardenne, M., Steinfelder, K., and Tuemmler, R., MA-Massenspektrographie organischer Substantzen, Springer Verlag, Berlin, 1971.
This paper was first presented at the 13th International Tribology Colloquium, Technische Akademie Esslingen, Germany
|