|
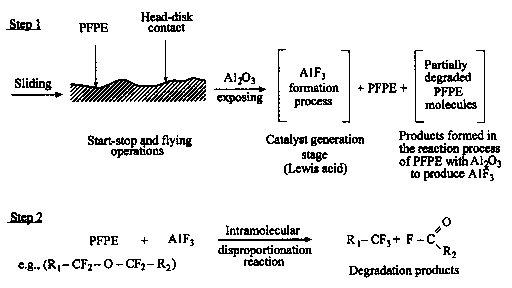 |
Fig. 14. Schematic presentation of the catalyzed PFPE degradation process.
|
The result of this comparison was concluded as follows: "What is most significantly revealed here is that the lubricant degradation in the disk environment is also singularly dominated by the Lewis acid catalyzed disproportionation reaction, and no other reaction of consequence is operative" (Kasai, 1999). Although the considered similarities are very interesting and might provide some evidence for the
alumina-catalyzed degradation mechanism of a Z-type lubricant, we will also show that another mechanism accounts for all the UHV-generated lubricant fragments (see subsection 5.2). Figure 14 schematically summarizes the catalytic degradation process of PFPE lubricants.
Recently, Fukuchi (1999) investigated the chemical degradation of major PFPE lubricant fluids, such as Z-DOL, Fomblin AM-2001, Z-25, Demnum SP-3, and Demnum SA, with bivalent metal ions. These lubricants were allowed to react with a variety of metal sulfates under identical heating conditions, and the resulting fluoride ion concentrations were measured. The respective reaction mechanisms involve an ether cleavage that is caused by the coordination of metal ions with oxygen atoms in the main chain of the PFPE. Each lubricant with a given sulfate was heated at 250 °C in an oven. Heating times were 30, 60, 90, or 150 min. In terms of the ability to degrade a lubricant, metal ions were evaluated in sequence from Cu+2 > Ni+2 > Co+2 > Fe+2 > Mn+2 > Mg+2. This trend was found to be explained by the ionization potentials of the metal atoms. For an identical metal ion, the extent of degradation was somewhat higher with Fomblin than with Demnum lubricants.
4.4. Electron-Induced Degradation Mechanism
It is well known that a wide variety of materials, after mechanical treatment, become electron emitters. The term "exoelectron emission" relates to low-energy electrons and originated from the investigation of the emission in freshly formed metals, which were accounted for as a consequence of an exothermal transformation process of the surface (Kramer, 1950). Figure 1 demonstrates that exoelectron emission occurs when a material's surface is disturbed by plastic deformation, abrasion, fatigue cracking, or phase transformation. The energy of exoelectrons is considered to be very low, of the order of 1-3 eV (Chaikin, 1967; Dickinson et al., 1997). Wei et al. (1998) reported that the energy of triboelectrons ranges form 0.5 to 80 eV, depending on the materials and test conditions. However, there is no reference given either to who measured the energy of these electrons or to the apparatus or technique used. There is also no information related to the mentioned materials and test conditions. Grunberg (1958) reviewed the exoelectron emission phenomena. Kajdas (1994) discussed the importance of low-energy electrons for the initiation of tribochemical reactions, proceeding according to the NIRAM approach. The principal thesis of the model is that lubricant molecules, for example, alcohols (Kajdas, 1987), form anions that are then chemisorbed on the positively charged areas of rubbing surfaces. Vurens et al. (1992) examined the bonding of the thin PFPE films by low-energy electrons and UV. They provided evidence that the bonding takes place through an interaction of the perfluoropolyether molecule, with a low-energy photoelectron emitted by excitation of the substrate by the UV photons, and demonstrated that for a Demnum S200 film on a diamondlike carbon substrate bombarded with low-energy electrons, the most abundant fragment ions comprise the following negative species: F¯, C3F5O2¯, and C3F7O¯. Therefore, the electron mediated degradation mechanism of perfluoropolyether lubricants seems to be of particular importance.
There is no reference that directly refers to triboemission of the head-disk interface. However, Nakayama, Yamanaka, et al. (1997) simulated the head-disk interface by using the friction system of alumina sliding on a-C:H films and found that both negatively charged particles and positively charged particles are emitted under friction in ambient air. Matsunuma et al. (1996), using empirical molecular orbital (MO), simulation, showed that an electron attachment to a PFPE model leaving a methylene oxide segment reduced the bond order of the C—O bond. Thus the cleavage of the weakest C—O bond of the anion radical produced an anion and a neutral radical easily. This is in line with the NIRAM approach (Kajdas, 1987; Kajdas, 1994). Previously, this approach was also successfully applied in accounting for the vinyl tribopolymerization process in both metal-on-metal systems (Kajdas et al., 1993) and ceramic-on-ceramic systems (Furey et al., 1997) Therefore, it is reasonable to suggest that anionic intermediates play an important role in the electron-induced degradation process of PFPE lubricants. A schematic presentation of the low-energy electron-induced PFPE degradation process is shown in Fig. 15.
4.5. Mechanical Degradation
The application of an adequate shear stress results in the ordering of the PFPE chain and a corresponding
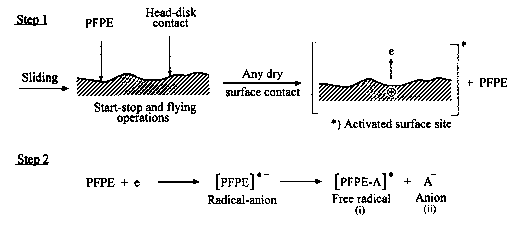 |
Fig. 15. Schematic showing the electron-induced PFPE degradation process:
- free radicals can recombine with other radicals, react with PFPE molecules, decompose, or interact with low-energy electrons to form other anions;
- anions react with the positively charged sites of the surface.
|
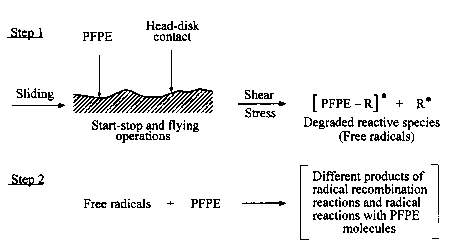 |
Fig. 16. PFPE degradation process caused by shearing.
|
decrease in friction (Hirz et al., 1992). Under operating conditions, the removal of PFPE lubricant molecules from sliding and flying tracks, comprises its displacement and loss. The removal rate depends on film thickness, chemical structure, and molecular weight. Microscale friction and properties of molecularly thin liquid films most recently were discussed in detail by Bhushan (1999b). In flying and sliding, the lubricant removal rate from monolayer films is significantly slower than from multilayer films (Novotny and Baldwinson, 1991). The lubricant loss in sliding can be due to polymer scission (Buchholz and Wilson, 1986). Direct mechanical scission at shear rates of 108-1010 s -1; is possible (Novotny and Baldwinson, 1991; Bhushan, 1999b); however, other factors can contribute to the lubricant degradation as well. Mechanical scission of PFPE bonds leads to the formation of free radicals, similarly as in the thermal degradation process. Figure 16 provides a schematic presentation of the PFPE degradation process caused by high shear stresses.
5. DEGRADATION OF PFPE LUBRICANTS UNDER SLIDING CONDITIONS
5.1. General Information
Under disk-drive operation conditions, the head slider slides on the disk surface during start and stop operations. CSS operations lead to elastic and plastic deformation of asperities and some wear. The generated energy impacts the lubricant molecules, which are both physically and chemically adsorbed. The adsorbed and chemically bonded PFPE molecules undergo important changes caused by physical and chemical processes. Physical changes include the mechanical displacement of the molecules and their possible desorption or evaporation. Chemical changes are much more complex and can proceed according to different mechanisms, as discussed in Section 4. The common denominator of all these specific mechanisms is the lubricant degradation process leading to its loss, mostly in the form of gaseous species generated at the sliding contacts. Currently, for detection of gaseous species generated by sliding in a high vacuum chamber, the most widely used measurement technique relates to quadrupole mass spectrometry. Data concerning the detection of gaseous wear species from lubricated thin-film disks have been presented by Vurens et al. (1992), Strom et al. (1993), Novotny et al. (1994), Bhushan and Ruan (1994), Bhushan and Cheng (1997), and Li and Bhushan (1997).
5.2. Discussion on PFPE Lubricant Degradation Mechanisms
Novotny et al. (1994) applied high-resolution mass spectrometry to monitor in situ gaseous wear products generated during the sliding of lubricated carbon-carbon interfaces in a vacuum. Lubricant loss, alteration, and removal as precursors of the wear of solids were determined by scanning microelipsometry. The amount of altered lubricant that was left on the disk and slider surfaces was quantified by X-ray photelectron spectroscopy (XPS), and the chemical structure of altered lubricant was evaluated by time-of-flight mass spectrometry. It was believed that the dominating process, before the wear of solids occurs, is the tribochemical scission of fluorocarbon polymers. In other words, the processes occurring at slider-disk interfaces take place before a microscopic wear of solids, excluding local microscopic wear at asperities. Novotny et al. (1994) also compared the mass spectra obtained during sliding experiments with those obtained in thermal desorption and found that the spectrum during sliding at 0.5 m/s resembled the thermal spectrum at 300 °C rather than that at 90 °C. Thus, they speculated that the loss mechanism of lubricants could be of two types: either transient interfacial temperatures were high enough to evaporate lubricant from the surface, or polymer scission produced fragments small enough to be gaseous. It is of note at this point, however, that according to the work by Bair et al. (1991) and Bhushan (1992), local increases in temperature at contact spots are less than 100 °C for typical test conditions. From the mass spectrum of wear fragments and thermal and electron decomposition products of PFPE lubricants, Vurens et al. (1992) concluded that the degradation of PFPE lubricants in sliding is an electron mediated process, since most of the product fragments generated by low-energy electrons were comparable with that in sliding experiments.
Li and Bhushan (1997) studied the degradation process of Z-DOL and Fomblin AM2001 lubricants on the thin-films disks with an Al2O3-TiC slider under high vacuum. Gaseous products generated from the head-disk interfaces were detected and monitored as a function of sliding distance by using a quadrupole mass spectrometer. They found that the gaseous products generated from these two lubricants are quite similar. Decomposition of both lubricants was found to take place from the beginning of sliding, and the evolution rate of these gases decreased with the sliding time. Surface roughness of the disk has an effect on the sliding distance to failure, but little effect on the decomposition of lubricant.
Using the same setup, Bhushan and Cheng (1997) investigated three kinds of lubricants with nonpolar and polar ends and with and without thermal treatment, which were applied over a thin-film disk with DLC overcoat. They found that fluorocarbon fragments are generated from lubricants during a period of sliding with a low and stable coefficient of friction, followed by a sharp rise in frictional force and generation of gaseous products of DLC overcoat material. It was concluded that decomposition of PFPE lubricants begins from the onset of sliding, and the DLC overcoat starts to wear simultaneously from the beginning of the sliding for untreated lubricants but was well protected in the case of chemically bonded lubricants. Detailed degradation mechanisms of PFPE lubricants are the most complex and arguable issue. For example, Wei et al. (1998) applied chemical bonding theory to analyze the degradation process.
Most recent high-vacuum test results (Zhao and Bhushan, 1999) demonstrate that the degradation process of the 1.5-nm-thick Z-DOL lubricant film generates CFO, HCF2, and CF species during sliding. CF2O
fragments have not been detected. Another extensive work (Zhao et al., 1999c) also includes the Z-DOL lubricant degradation process and clearly shows the importance of both CFO and CF2O species along with HCF2, CF3, and other fragments. The formed Z-DOL degradation fragments are accounted for in terms of the electron-induced degradation mechanism. For example, to account for the CF2H and CFCF3 Z-DOL degradation fragments detected in mass spectra under UHV sliding conditions, the following reactions are proposed (Zhao et al., 1999c):
—CF2—CF2—O—CF2—O—CF2— + e →
—CF2—CF2—O¯ + •CF2—O—CF2 |
A¯ (anion) R• -(free radical)
| (1)
|
The negative ion A¯ reacts with the DLC surface. The formed free radical R• can further react in various ways. Some reactions follow, which lead to the formation of two species HCF2and CF3 found in mass spectra:
R• + H• → HCF2—O—CF2—
Compound I
| (2) |
R• + Z-DOL → CF3—O—CF2— + [Z—DOL—F]•
Compound II
| (3)
| | |
Compounds I and II, interacting with emitted low-energy electrons, generate the fragments detected in mass spectra in the following reactions:
HCF2—O—CF2— + e → •CF2H + ¯O—CF2—
| (4) |
CF3—O—CF2— + e → •CF3 + ¯O—CF2—
| (5)
|
Negative ions formed in reactions (4) and (5) react with the DLC positively charged spots generated during sliding. There are several possibilities to produce CF and CF2 fragments during the Z-DOL degradation process. One possibility to generate CF2 species may relate to the decomposition of CF2CF2O species into CF2 and CF2O by friction shear as well as electron impact (Wei et al., 1998). CF2 may be further cleaved into the F atom and CF species.
The detailed Z-DOL lubricant degradation mechanism, as described by Zhao et al. (1999c), emphasizes the importance of the negative-ion-radical action mechanism for the PFPE lubricant degradation process. The experimental results of that study also show that the decomposition of the lubricant molecule is greatly affected by the mechanical shearing. The Z-DOL lubricant molecule presents a linear long chain structure, including mostly weaker C—O and C—C bonds; the lubricant molecules are easily subjected to cutting by the microasperities on the rubbing surfaces, which results in the decomposition of the lubricant in sliding process. No obvious effect of temperature on the decomposition of the lubricant was observed in the test described by Zhao et al. (1999c).
Step 1 Low-energy electron emission process (1-4 eV) during sliding

Step 2 Attachment of emitted electron to lubricant molecule
Z-DOL + e → —CF2—CH2O¯ + H•
(Reactive species)
Step 3 Chemical reactions with the surface
Bonded molecule
H• species may recombine and generate hydrogen molecules H2
or they can interact with the dangling bonds
Bonded hydrogen atom
Fig. 17. Interaction of emitted electrons with Z-DOL lubricant molecules and subsequent interactions with the surface (Zhao et. al., 1999b).
| | | | |
Interestingly, the NIRAM approach can also be applied in accounting for experimental results concerning chemical bonding of PFPE lubricant films with DLC under sliding conditions. The most recent
research (Zhao et al., 1999b) demonstrated how low-energy electrons may attach to the PFPE lubricant molecules and allow the chemical reactions between lubricant molecules and a DLC surface. Figure 17 depicts the steps through which lubricant Z-DOL molecules are chemically bonded to the DLC surface after sliding. Under sliding conditions, low-energy electrons are emitted at the interface (Step 1); these electrons then attach to lubricant molecules forming reactive species (Step 2). The generated negative ions ( —CF2—CH2O¯) react with the surface and form bonded molecules (Step 3). Hydrogen atoms (free radicals) may recombine and produce hydrogen molecules H2, or they can interact with dangling bonds on the surface. Figure 18 describes the steps through which lubricant Z-15 or Z-25 molecules are chemically bonded to the DLC surface after sliding. Step 1 is similar to that in Fig. 17. The low-energy electrons then attach to lubricant molecules and form the reactive species (Step 2). The produced negative-ion reactive species allow chemical reaction with the surface and form bonded molecules; free-radical species can react with the surface dangling bonds (Step 3a). Free radicals can also recombine, forming a PFPE molecule, or they can interact with Z-15 lubricant molecules, producing a smaller PFPE compound and generating another free radical [Z-15-F}•. The latter radical can recombine with •CF2- free radical, generating a higher molecular-weight lubricant compound. More research is needed, however, to better understand all these processes.
Step 1 Low-energy electron emission process (1-4 eV) during sliding

Step 2 Attachment of emitted electron to lubricant molecule
—CF2—O—CF2— + e → —CF2—O¯ +•CF2—
Step 3 Further Chemical reactions a) with the surface
Bonded molecule
Bonded molecule
b) between lubricant fragments
•CF2— + •CF2— → —F2C—CF2—
Z15 + •CF2— → [Z-15—F]• + CF3—
| | | |
|
Fig. 18. Interaction of emitted electrons with Z-15 or Z-25 lubricant molecules and subsequent interactions with the surface (Zhao et al., 1999b).
|
6. CONCLUDING REMARKS
This review paper is mostly focused on interaction and degradation mechanisms of PFPE lubricants with DLC protective overcoats for magnetic media. Major stress is put on the degradation process of these lubricants under sliding conditions. A detailed discussion of various degradation mechanisms of Z-DOL lubricant is provided, which includes thermal decomposition, catalytic degradation, electron-mediated degradation, and mechanical degradation processes. Comparing various degradation mechanisms, we suggest that the catalytic degradation mechanism proposed by other authors is not relevant, because kinetics is slow at asperity temperatures. Based on a wide variety of reviewed or discussed experimental data, it is reasonable to emphasize that anionic intermediates (negative ions and/or negative-ion-radical species) produced by low-energy electrons play an important part in both
- the electron-mediated degradation process of PFPE lubricants, and
- chemical bonding of PFPE lubricant films with DLC surfaces under sliding conditions.
This is in line with the NIRAM approach.
ACKNOWLEDGMENT
The authors acknowledge Dr. Zheming Zhao for his help in figure preparation and for fruitful discussions. Financial support for this research was provided by the industrial membership of the Computer Microtribology and Contamination Laboratory.
REFERENCES
- Bair, S., Green, L., and Bhushan, B. (1991), "Measurement of Asperity Temperatures of a Read/Write Head Slider Bearing in Hard Magnetic Recording Disks," ASME J. Tribol. 113, 547-554.
- Bhushan, B. (1992), "Magnetic Head-Media Interface Temperatures. Part 3: Applications to Rigid Disks," ASME J. Tribol., 114, 420-430.
- Bhushan, B. (1994), "Tribology of Magnetic Storage Systems," in CRC Handbook of Lubrication and Tribology (E. R. Booser, ed.), Vol. III, pp. 325-371, CRC Press, Boca Raton, Florida.
- Bhushan, B. (1996), Tribology and Mechanics of Magnetic Storage Devices, 2nd ed., Springer-Verlag, New York.
- Bhushan, B. (1999a), "Chemical, Mechanical and Tribological Characterization of Ultra-Thin and Hard Amorphous Carbon Coatings as Thin as 3.5 nm: Recent Developments," Diamond and Related Materials, 8, 1985-2015.
- Bhushan, B. (1999b), Principles and Applications of Tribology. Chap. 11, Wiley, New York (Micro/Nanotribology).
- Bhushan, B. and Cheng, Y. (1997), "Wear and Degradation Mechanisms of Magnetic Thin Film Rigid Disks with Different Lubricants Using Mass Spectrometry," J. Appl. Phys., 81, 5390-5392.
- Bhushan, B. andRuan,). (1994), "Tribological Performances ol Thin Film Amorphous Carbon Overcoats for Magnetic Recording Disks in Various Environments," Surface Coating Technol., 68/69, 644-650.
- Bhushan, B. and Zhao, Z. (1999), "Macroscale and Microscale Tribological Studies of Moleculary Thick Boundary Layers of Perfluoropolyether Lubricants for Magnetic Thin-Film Rigid Disks." J. Info. Storage Proc. Syst., 1, 1-21.
- Bonder, G. M. and Pardue, H. L. (1989), Chemistry-An Experimental Science, Wiley, New York.
- Buchholz, F. L. and Wilson, L. R. (1986), "High Shear Rheology and Shear Degradation of Aqueous Polymer Solutions," J. Appl. Poly. Sci., 32, 5399-5413.
- Chaikin, S. W. (1967), "On Frictional Polymer," Wear-10, 49-60.
- Cho, N. H., Krishnan, K. M., Veirs, D. K., Ruhin, M. D.. Hopper, C. B., Bhushan, B., and Bogy, D. B. (1990), "Chemical Structure and Physical Properties of Diamond-Like Amorphous Carbon Films Prepared by Magnetron Sputtering," J. Mat. Res., 5, 2543-2554.
- Del Pesco, T. W. (1994), "Perfluoroalkylpolyethers" in CRS Handbook of Lubricant and Tribology (E. R. Booser, ed.), Vol. Ill pp. 287-303, CRC Press, Inc. Boca Raton, Florida.
- Dickinson, J. T, Scudiero, L., Yasuda, K., Kim, M-W., and Langford, S. C. (1997), "Dynamic Tribological Probes: Particle Emission and Transient Electric Measurements," Tribol. Lett., 3, 55-67.
- Dillon, R. 0., Woolman, J. A., and Katkanant, V. (1984), "Use of Raman Scattering to Investigate Disorder and Crystallite Formation in As-Deposited and Annealed Carbon Films," Phys. Rev. B29, 3482-3489.
- Enke, K. (1981), "Some New Results on the Fabrication of and the Mechanical, Electrical and Optical Properties of i-Carbon Layers." Thin Solid Films 80, 227-234.
- Fukuchi, T. (1999), "Degradation of Perfluoropolyether Fluids with Metal Ions," ASME J. Tribol. 121, 348-351.
- Furey, M. J., Kajdas, C., Kempinski, R., and Tripathy, B. S. (1997), "Tribochemistry of Vinyl Monomers Under Boundary Lubrication of Alumina-on-Alumina System," Lubr. Sci. 10, 3-25.
- Grunberg, L. (1958), "A Survey of Exoelectron Emission Phenomena," Br. J. Appl. Phys. 9, 85-93.
- Gschwender, L. ).. Snyder, C. E. and Fultz, G. W. (1992). "Soluble Additives for Perfluoropolyalkylether Liquid Lubricants." Lub. Eng. 49, 702-708.
- Gumprecht, W. H. (1966), "A New Class of High-Temperature Fluids," ASLE Trans. 9, 24-30.
- Hara, H., Kubota, M., Enolo, I., and Ohmae, N. (1991), "Effect of Lubricant Durability of Thin Film Disk Media," Adv. Info. Storage Syst. 2, 587-592.
- Heideman, R. and Wirth, M. (1984), "Transforming the Lubricant on a Magnetic Disk into a Solid Fluorine Compound," IBM Tecb. Disclosure Bull. 27, 3199-3205.
- Helmick, L. S. and Jones, W. R. (1990), "Deformation of the Thermal Stability of Perfluoroalkylethers," NASA memo TM-102493, NASA, Washington, D.C.
- Hirz, S. I., Homola, A. M., Hadziiannou, G., and Frank, C. W. (1992), "Effect of Substrate on Shearing Properties of Ultrathin Polymer Films," Langmuir, 8, 328-333.
- Homola, A. M., Lin, L.}. and Saperstein, D. D. (1990), "Process for Bonding Lubricant to a Thin Magnetic Recording Disk," U.S. Patent 4,960,609, October 2.
- Huu, T. Le, Zaidi, H. and Paulmier, D. (1997), "Friction and Wear Properties of Hard Carbon Coatings at High Sliding Speed," Wear 203-204, 442-446.
- Jansen, F., Machonkin, M., Kaplan, S., and Mark, S. (1985), "The Effects of Hydrogenation of the Properties of Ion Beam SputterDeposited Amorphous Carbon," J. Vac. Sci. Technol. A3, 605-609.
- Janzen J. (1982), "Physichemical Characterization of Carbon Black," Chem. Technol. 55, 669-706.
- Jones, W. R., Jr. (1995), "Properties of Perfluoropolyethers for Space Applications," Tribol. Trans. 38, 557-564.
- Jones, W. R., Paciorek, K. J. L., Ito, T. I., and Kratzer, R. H. (1983), "Thermal Oxidative Degradation Reactions of Linear Perfluoroalkyl Ethers," Ind. Eng. Chem. Prod. Res. Dev. 22, 167-170.
- Jones, W. R., Jr. and Snyder, C. E., Jr. (1980), "Boundary Lubrication, Thermal and Oxidative Stability of A Fluorinated Polyether and Perfluoropolyether Triazene," ASLE Trans. 23, 253-261.
- Kajdas, C. (1987), "About an Anionic-Radical Concept of The Lubrication Mechanism of Alcohols," Wear 116, 167-180.
- Kajdas, C. (1994), "Importance of Ionic Reactive Intermediates For Lubricant Component Reactions With Friction Surfaces," Lubr. Sci. 6, 203-228.
- Kajdas, C. (1997), "Industrial Lubricants," in: Chemistry and Technology of Lubricants R. M. Mortier and S. T. Orszulik (eds.), Chap. VIII, Blackie Academic & Professional, London.
- Kajdas, C., Lafleche, P. M., Furey, M. J., Hellgeth, J. W., and Ward, T.C. (1993), "A Study of Tribopolymerization under Fretting Contact Conditions," Lubr. Sci. 6, 51-89.
- Kaplan, S., Jansen, F., and Machonkin, M. (1985), "Characterization of Amorphous Carbon-Hydrogen Films by Solid-State Nuclear Magnetic Resonance," Appl. Phys. Lett. 47, 750-753.
- Kasai, P. H. (1992). "Perfluoropolyethers: "Intramolecular Disproportionation," Macromolecules 25, pp. 6791-6799.
- Kasai, P. H. (1999), "Degradation of Perfluoropolyethers and Role of X-1P Additives in Disk Files," J. Info. Storage Proc. Syst. 1, 22-31.
- Kasai, P. H., Tang, W. T, and Wheeler, P. (1991), "Degradation of Perfluoropolyethers Catalyzed by Alumina Oxide," Appl. Surf. Sci. 51, 201-211.
- Kramer, J. (1950), Der metallische Zustand, Vandenhock and Ruprecht, Goettingen, Germany.
- Lee, T. D. (1991), "Enhanced Chemical Bonding Between Lubricants and Magnetic Coatings by High Energy Irradiation and its Application to Tape Durability," J. Vac. Sci. Technol. A9, 1287-1292.
- Lee, J. K., Smallen, M,, Enguero, J., Lee, H. J., and Chao, A. (1993). "The Effect of Chemical and Surface Properties of Hydrogenerated Carbon Overcoats on the Tribological Performance of Rigid Magnetic Disks," IEEE Trans. Magnet. 29, 276-281.
- Lee, H. J., Zube, D., Hollar, D., Lee, J. K., Chao, A., and Smalinn, M. (1993), "Enhanced Tribological Performance of Rigid Disk by Using Chemically Bonded Lubricant," J. Vac. Sci. Technol. A13, 711-714.
- Li, Y. and Bhushan., B. (1997), "Degradation of Perfluoropolyether Lubricants on Magnetic Recording Disks During Sliding," Proc. Inst. Mech. Eng. Part J, 211, 279-288.
- Matsunuma, S., Miura, T., and Kataoka, H. (1996), "Theoretical Simulations of Electron-Induced Degradation of Perfluoropolyether," Tribol. Trans. 39, 380-385.
- Miller, D. J. and McKenzie, D. R. (1983), "Electron Spin Resonance Study of Amorphous Hydrogenated Carbon Films," Thin Solid Films 108, 257-264.
- Nakayama, K., Bou-Said, B., and Ikeda, H. (1997), "Tribo-Electromagnetic Phenomena of Hydrogenated Carbon Films: Tribo-Electrons, -Ions, -Photons, and -Charging," ASME ]. Tribol. 119, 764-768.
- Nakayama, K., Yamanaka, K., Ikeda, H., and Sato, T. (1997), "Friction, Wear, and Triboelectron Emission of Hydrogenated Amorphous Carbon Films," Tribol. Trans. 40, 507-513.
- Nakayama, K. and Ikeda, H. (1996), "Triboemission Characteristics of Electrons During Wear of Amorphous Carbon and Hydrogeneted Amorphous Carbon Films in A Dry Air Atmosphere," Wear 198, 71-76.
- Novotny, V. J. and Baldwinson, M. A. (1991), "Lubricant Dynamics in Sliding and Flying," J. Appl. Phys. 70, 5647-5652.
- Novotny, V. J., Pan, X., and Bhatia, C. S. (1994), "Tribochemistry at Lubricant Interfaces," J. Vac. Sci. Technol. A12, 2879-2886.
- Perry, S. S. and Somorjai, G. A. (1995), "The Interaction of Short-Chain Model Lubricants with the Surfaces of Hydrogenated Amorphous Carbon Films," Tribol. Lett. 1, 47-58.
- Saperstein, D. and Lin, L. (1990), "Improved Surface Adhesion and Coverage of Perfluoropolyether Lubricants Following for-UV Irradation," Langmuir 6, 1522-1524.
- Silva, S. R. P., Amaratunga, A. J., and Constantinou, C. P. (1992), "Optical Properties of Amorphous Carbon/Diamond Thin Films." J. Appl. Phys. 72, 1149-1153.
- Simonds, J. L. (1995), "Magnetoelectronics Today and Tomorrow," Phys. Today April, 6-32.
- Strom, B. D., Bogy, D. B., Walmsley, R. G., and Brandt, J. (1993), "Gaseous Wear Products from Lubricated Thin Disk," IEEE Trann. Magnet MAG-29, 253-258.
- Torng., C. J. and Silvertsen, J. M. (1990), "Structure and Bonding Studies of the C:N Thin Films Produced by rf Sputtering Method." J. Mat. Res. 5, 2490-2496.
- Vurens, G. H., Gudeman, C. S., Lin, L. J., and Foster, J. S. (1992), "Mechanism of Ultraviolet and Electron Bonding of PFPEs," Langmuir 8, 1165-1169.
- Wada, N., Gaczi, P. J., and Solin, S. A. (1980), "Diamond-like Threefold Coordinated Amorphous Carbon," J. Non-Cryst. Solid 35, 543-548.
- Waltman, R. J., Pocker, D. J., and Tyndall, G. W. (1998), "Studies on the Interactions between Z-DOL Perfluoropolyether Lubricant and The Carbon Overcoat of Rigid Magnetic Media," Tribol. Lett. 4, 267-275.
- Wang, R., Meeks, S. W., White, R. L., and Weresin, W. E. (1995). "The Effect of Hydrogen in Carbon Overcoats on The Tribology of The Head-Disk Interface," IEEE Trans. Magnet. 31, 2919-2921.
- Wang, R. H., White, R. L., Meeks, S. W., Min, B. G., Kellock, A., Homola, A., and Yoon, D. (1996), "Interaction of Perfluoropolyether with Hydrogenated Carbon," IEEE Trans. Magnet. 32, 3777-3779.
- Wei, J., Fong, W., Bogy, D. B., and Bhatia, C. S. (1998), "The Decomposition Mechanisms of a Perfluoro-polyether at the Head/Disk Interface of Hard Disk Drives," Tribol. Lett. 5, 203-209.
- Yanagisawa, M. (1994), "Adsorption of Perfluoro-Polyethers on Carbon Surfaces," in Tribology and Mechanics of Magnetic Storage Systems, Vol. 9, SP-36, 25-32, STLE, Park Ridge, Illinois.
- Zehe, M. J. and Faut, 0. D. (1989), "Acidic Attack of Perfluorinated Alkyl Ether Lubricant Molecules by Metal Oxide Surface," NASA Memo TM-101962, NASA, Washington, D.C.
- Zhao, Z. and Bhushan, B. (1996), "Effect of Bonded Lubricant Films on the Tribological Performance of Magnetic Thin-Film Rigid Disks," Wear 202, 50-59.
- Zhao, Z. and Bhushan, B. (1999), "Tribological Performance of PFPE and X-1P Lubricants at Head-Disk Interface. Part I. Experimental Results," Tribol. Lett. 6, 129-139.
- Zhao, Z., Bhushan, B., and Kajdas, C. (1999a), "Tribological Performance of PFPE and X-1P Lubricants at Head-Disk Interface. Part II. Mechanisms," Tribol. Lett. 6, 141-148.
- Zhao, Z., Bhushan, B., and Kajdas, C. (1999b), "Effects of Thermal Treatment and Sliding on Chemical Bonding of PFPE Lubricant Films with DLC Surfaces," J. Info. Storage Proc. Syst. 1, 259-264.
- Zhao, X., Bhushan, B., and Kajdas, C. (1999c), "Lubrication Studies of Head-Disk Interfaces in a Controlled Environment: Part II. Degradation Mechanisms of PFPE Lubricants," Proc. Inst. Mech. Eng., Part J (in press).
|