|
Triboemission as a Basic Part of the Boundary Friction Regime: A Review
C. Kajdas
Warsaw University of Technology, Plock, and Central Petroleum Laboratory, Warsaw, Poland, and
M.J. Furey, A.L. Ritter, and G.J. Molina
Virginia Polytechnic Institute and State University, Blacksburg, Virginia, USA
Abstract
This paper reviews selected phenomena related to the boundary friction process. The central thrust is on triboemission, defined as emission of electrons, charged particles, photons, etc., under conditions of boundary friction and I or surface damage caused by fracture processes. Low-energy electrons are one of the most important particle components in triboemission. Accordingly, the triboemission process is of particular significance for (i) the boundary friction process as such, and (it) the tribochemistry of the boundary lubrication process. This review considers all the major issues of triboemission and. related phenomena I processes. It is shown that, in the boundary lubrication regime with hydrocarbon lubricants, charge intensities decrease with an increase in lubricant molecular weight. The paper also includes some recent information concerning research on triboemission along with a newly developed apparatus.
Keywords: triboemission, boundary friction, boundary lubrication, electron emission, tribochemistry
INTRODUCTION
The nature and the consequences of the interactions that take place at a tribological contact are associated with a wide variety of physical processes and also control the friction and wear behaviour of the materials involved. During these interactions: forces are transmitted; energy is exchanged; the physical and chemical natures of the materials are changed; the surface topography is altered; and sometimes loose wear particles are generated. All these seemingly random and complex surface phenomena follow a certain order and satisfy the laws of nature, as explained elsewhere [1].
Wear of materials occurs by many different mechanisms, depending on the materials, the environment, the operating conditions, and the geometry of the wearing bodies. These wear mechanisms can be classified into two groups:[1] (i) those dominated primarily by the
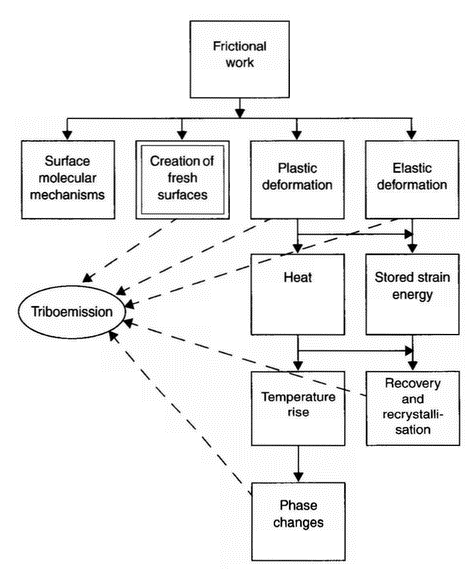 |
Figure 1: Schematic representation of energy dissipation [3] |
mechanical behaviour of solids and (ii) those dominated primarily by the chemical behaviour of materials. What determines the dominant wear behaviour are the mechanical properties, the chemical stability of the materials, the operating conditions, and the environment [2]. As an example, Figure 1 illustrates the most likely primary energy-absorbing mechanisms during abrasive wear and some resulting secondary dissipation processes. About 90% of the work done in plastically deforming material during abrasion is converted to heat, which may subsequently cause local temperature rises and phase changes, and can provide the thermal activation for recovery and recrystallisation processes [3]. The application of mechanical energy by friction to
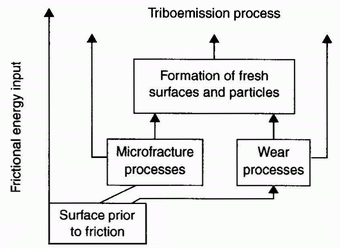 |
Figure 2: Physical processes associated with surface enlargement during friction [2] |
enlarge a solid surface may give rise to a great number of physical processes (Figures 1 and 2). The surface enlargement clearly creates new surfaces, which form a bridge between the physics and chemistry of wear processes, leading to microscopic aspects of a wide variety of wear mechanisms. These mechanisms are often connected with tribochemical reactions that are initiated by the effects of surface-enlargement.
Typical physical phenomena resulting from wear processes under the boundary friction regime encompass mostly high temperatures and triboemission. The central thrust of the present paper is to discuss triboemission processes along with the possible influence of the emitted particles on mechanochemistry. Mechanochemistry is a branch of chemistry dealing with the chemical and physicochemical changes of substances of all states of aggregation due to the influence of mechanical energy [4]. Mechanical influence plays a significant role with regard to chemical reactions where at least one solid component is involved. The most important reactions take place during the boundary lubrication process. Tribochemistry deals with these changes in solids [4]. As low-energy electrons are of particular importance in initiating tribochemical reactions [5], emphasis will be put on these exoelectrons.
Triboemission - a general description
Figure 2 is a schematic representation of the emission of electrons, photons, negatively and positively charged ions, phonons, X-rays, and gases due to the exposure of a fresh surface as a consequence of friction. Accordingly, triboemission may be defined as the emission of
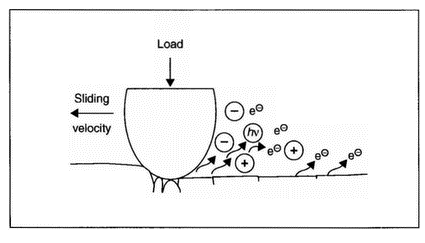 |
Figure 3: Conceptual view of triboemission [6] |
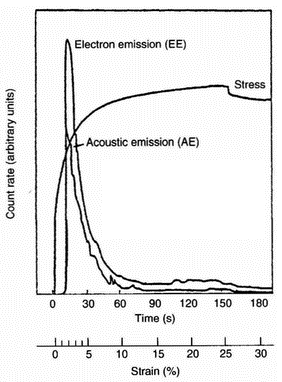 |
Figure 4: Acoustic emission, exoelectron emission, and stress vs. strain or time, for a sample of anodised Al 13450 [7] |
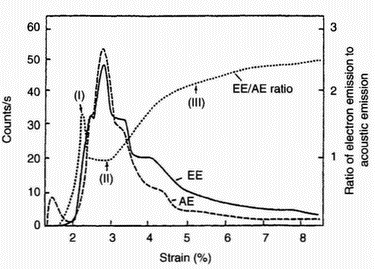 |
Figure 5: Electron emission (EE) and acoustic emission (AE) rates and their ratio (EE/AE) as a function of strain for a sample of anodised aluminium [7] |
these species (including acoustic emission) under conditions of tribological surface damage. An illustrative example of the triboemission process is shown in Figure 3 [6]. It has been shown [7] that during the tensile strain of anodised aluminium there are bursts of acoustic emission, closely associated with cracking of the oxide, that acoustic emission and electron emission count rates versus strain are closely related, and that most electron emission occurs very soon after acoustic emission. Both curves obtained simultaneously with two countrate meters are presented in Figure 4. Typical electron emission (EE), acoustic emission (AE), and EE/AE curves are shown in Figure 5. During the initial rise of the major peak (I), near 2% strain, the cracking of the oxide appears to be noticeably more efficient in generating electrons. Interestingly, optical microscopy showed [7] that there were initial cracks formed in the oxide, whereas the cracking occurring later was primarily crack extension. In the main portion of the emission curves (II) the ratio is near unity and constant. Finally, the EE/AE ratio climbs (III) as the AE curve is seen to drop off faster than the EE curve. Previous work [8] discusses the emission of neutral particles from oxide-metal systems. An example of the relationship between neutral emission and thickness is illustrated in Figure 6. The total neutral emission curve shows that the neutral
emission saturates at about 2500 A nominal thickness, in contrast to electron emission, which continues to climb at an exponential rate with thickness [8]. However, the ratio of oxygen emission to total
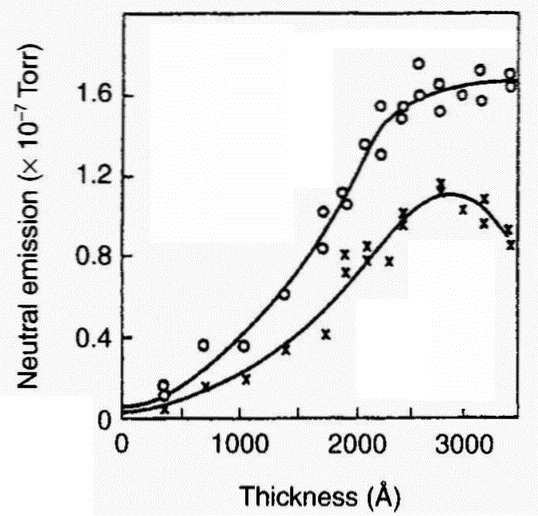 |
Figure 6: Neutral emission intensity vs. thickness for a dense oxide of bare 2024 Al: x = peak heights of 02; emission; o = peak heights of the total emission curves [8] |
emission or to total neutral emission - as can be seen in Figure 6 - decreases with increasing oxide thickness.
The present paper discusses only the emission of charged particles. Electron emission and negatively and positively charged particles are reviewed. A summary of fractoemission findings, including the material system and atmosphere, the measurement system, and reported emission level, is also given. First we will briefly discuss the nature of materials used in tribology.
CHARACTERISTICS OF THE SOLID MATERIALS USED IN TRIBOLOGY
Materials used in tribological applications tend to be the common materials typically used for engineering applications, which can be separated into those that are crystalline and those that are amorphous. They include metals (steels, copper-based bearing materials, soft metal bearing materials, and cast iron), ceramics and special alloys, graphite, diamond, polymeric materials, composites, cermets, etc. The mechanical and physical properties of the most important materials used in tribology are well described elsewhere [9]. The characteristics of solids that relate to both triboemission and mechanochemistry are summarised below.
All solids are composed of atoms or molecules. A crystalline material is made up of unit cells containing the repeat unit of atoms and molecules. The atomic or molecular units are bound by cohesive energy. The cohesive or binding energies for selected elements - along with their electronegativities (EN) and work functions - are given in Table 1. Refractory metals, such as tungsten, have a higher binding energy, 8.66 eV, than lower melting point metals, such as iron and
Table 1. Electronegativity work function and cohesive energy of selected elements |
I A | II A | |
3 1.0 Li 1.65 2.4 | 4 1.5 Be 3.33 | 13¬atomic number electronegativity, eV ®Al 3.34 ¬cohesive energy eV work function, eV ®4.3
11 0.9 Na 1.13 2.35 | 12 1.2 Mg 1.53 | IVB | VB | VIB | VIIB | |
19 0.8 K 0.94 2.2 | 20 1.0 Ca 1.83 2.8 | 22 1.6Ti 4.86 3.95 | 23 1.7 V 5.30 4.1 | 24 1.6 Cr 4.10 4.6 | 25 1.5 Mn 2.98 3.8 | 26 1.7 Fe 4.29 4.3 |
37 0.8 Rb 0.86 2.2 | 38 1.0 Sr 2.35 | 40 1.5 Zr 6.32 3.9 | 41 1.7 Nb 7.47 4.0 | 42 1.6 Mo 6.81 4.3 | 43 1.9 Tc
| 44 2.0 Ru 6.62 4.6 |
55 0.75 Cs 0.83 1.8 | 56 0.9 Ba 1.86 2.5 | | | 74 1.9 W 8.66 4.5 | 75 2.1 Re 8.10 5.0 | 76 2.0 Os 4.7 |
VIIIB | IB | IIB |
III A | IV A | |
5 2.0 B 5.81 4.5 | 6 2.5 C 7.36 4.7 |
13 1.5 AI 3.34 4.3 | 14 1.8 Si 4.64 4.8 |
27 1.7 Co 4.39 4.4 | 28 1.8 Ni 4.44 4.5 | 29 1.9 Cu 3.50 4.4 | 30 1.5 Zn 1.35 4.2 | 31 1.5 Ga 2.78 4.0 | 32 1.8 Ge 3.87 4.8 |
45 2.1 Rh 5.75 4.75 | 46 2.0 Pd 3.94 4.8 | 47 1.8 Ag 2.96 4.3 | 48 1.5 Cd 1.16 4.1 | 49 1.5 In 2.6 3.8 | 50 1.8 Sn 3.12 4.4 |
77 2.1 lr 6.93 5.3 | 78 2.1 Pt 5.85 5.3 | 79 2.3 Au 3.78 4.3 | | 81 1.7 TI 1.87 3.7 | 82 1.7 Pb 2.04 4.0 | |
copper, with binding energies of 4.29 and 3.5 eV, respectively. The binding energy of surface atoms is somewhat less than that, of bulk atoms because of the reduced number of near-neighbour interactions [10]. The periodic arrangement of atoms and the resulting periodicity of the electron potential energy gives rise to characteristic energy bands [11]. The different properties of metals, semiconductors, and insulators are determined by these energy bands, but often can be understood more easily in terms of near-neighbour chemical interactions. The properties of surfaces are usually different from bulk properties, and govern the tribological behaviour of a solid.
Ceramics are materials that are combinations of one or more metals with non-metallic elements, such as nitrogen, oxygen, or carbon. The bulk free energy of formation of ceramics is very low, and, therefore, the bulk crystals are very stable. However, the free energy of surface states can be much higher than the bulk free energy so that under boundary lubrication conditions the surface can be quite reactive. The ceramic atoms are held together mostly by covalent bonding, while some ceramics have ionic bonding.
An amorphous material does not have long-range order and the concomitant periodic potential, but does exhibit some of the same basic electrical and optical properties as a crystalline material, because they are based on the properties of chemical bonds rather than uniquely on long-range order. Accordingly, some of the most basic optical and electrical properties of both crystalline and amorphous solids can be described quantitatively by considering the nature of the chemical bonds between atoms rather than the effect of a periodic potential. The band gap between the valence and conduction bands of ionic solids is generally larger than that in covalent solids (diamond has an exceptionally large gap for a covalent solid). The largest band gap for any material is DE = 11.5 eV in the ionic solid lithium fluoride, whereas the band gap in the covalently bonded semiconductor silicon is DE =1.1 eV [12]. Metals have partially occupied bands so display freeelectron behaviour with large electrical conductivity. The electrical conductivity of various solids varies from ~108 (W m) -1 for metals to less than 10 -14 (W m) -1 for insulators. Semiconductors usually have a room temperature conductivity of the order of 102 (W m) -1, although this value is strongly dependent on both the temperature and the purity of the semiconductor. From the viewpoint of both electron emission and electron attachment, electronegativity is important because it provides a scale for the affinity of an electron to a bond. Fluorine has the highest electronegativity and lithium the lowest, thus lithium fluoride has the largest energy gap.
TRIBOEMISSION RELATED TERMINOLOGY
Any electron emission process is the ejection of electrons from a substance into vacuum as a consequence of excitation by some external source of energy. Due to the fact that all substances are built up of atoms and all atoms comprise electrons, any solid may emit electrons from its surface. The process is analogous to that ofionisation of a free atom. As the energy of electrons in an atom is lower than that of an electron at rest in vacuum, some energy must be added to the electrons to release them. The term 'exoelectron emission' (Kramer effect)
Table 2. Terminology related to the major charged particle emission processes* |
Emission process | Most used abbreviations | Abbreviations proposed | Notes |
Exoelectron emission (exoemission) | EEE, EE | EE | Primary excitation |
Triboemission | TE | TRE |
Fractoemission | FE | FRE |
Thermally stimulated exoelectron emission | TSEE | TSE | Primary excitation plus secondary excitation with stimulus |
Photo-stimulated exoelectron emission | PSEE | PSE |
Optically stimulated exoelectron emission | OSEE |
Photoexoelectron emission | PEEE |
Field stimulated exoelectron emission | FSEE | FSE |
Chemisorptive exoelectron emission | CSEE | CSE |
*Modification of the table on exoelectron terminology in Ohmae et.al.[14].
originates from investigation of the emission in freshly treated metals (see below, p. 233). Exoelectrons are electrons of low energy spontaneously emitted from most fresh surfaces, or are electrons emitted from surface atoms under certain conditions that provide enough energy to provoke their evaporation. It turns out that such conditions are closely correlated with the surface changes produced by wear cracks and material fatigue in general [13]. Exoelectron emission is also defined as transitory low-energy electron emission by substances after excitation of some kind (called primary excitation), after spontaneous stimulation, or due to the input of energy that is less than the work function of substances (called secondary excitation).
Table 2 presents the terminology related to exoelectron emission, triboemission, and fractoemission. The exoelectron emissions are named according to the type of secondary excitation stimuli, but, for triboemission and fractoemission, the stimuli are not indicated because both tribo- and fractoemission phenomena include the emission not only of electrons but also of positively and negatively charged particles, neutral particles, gases, photons, phonons, and even X-ray emission.
Vacuum level
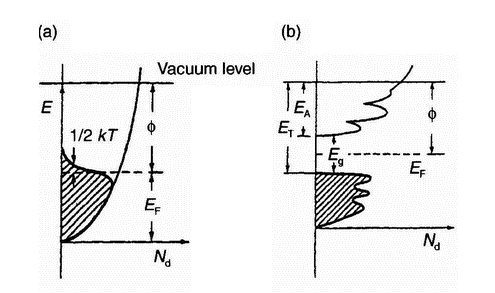 |
Figure 7: Density of occupied and empty states in (a) a metal and (b) a semiconductor or insulator [12]
EF = Fermi level
f = work function
EA = electron affinity
ET = photoelectric threshold
Eg = fundamental gap
for metal: ET = f |
EXOELECTRON EMISSION (EXOEMISSION): Conventional electron emission
Conventional electron emission includes (i) thermionic emission, (ii) photoemission, and (iii) field emission, corresponding to different energy sources, thermal, photons, and external electric fields, for ejecting electrons from a solid. These processes are reviewed before a discussion of the less well-understood exoelectron emission.
Thermionic emission occurs when the temperature of a material is high enough such that kT is of the order of the work function f of the material (T is the absolute temperature and k is the Boltzmann constant). The emission current is given by
/ = KT 2exp(-f/kT)
where K is a characteristic constant for the material. The main factor controlling the change of current with temperature is the exponential term. Since exp(f/k) is of the order of 105 K for typical metals, very high temperatures are necessary to obtain significant emission currents.
Photoemission occurs when an electron absorbs a photon with energy greater than the work function of the material. The photoelectric effect is a quantum phenomenon where the maximum kinetic energy of the emitted electrons is ( hn - f ). Figure 7 is an energy-band diagram for photoemission from a clean metal surface [15]. EF is the Fermi level separating the highest-energy filled electron states from a continuum of higher-lying unfilled states.
Field emission is the ejection of electrons from a solid due to an external electric field. The potential energy of an electron near the surface of a metal is shown in Figure 8. The potential energy of an electron outside the metal is a linearly decreasing function of position
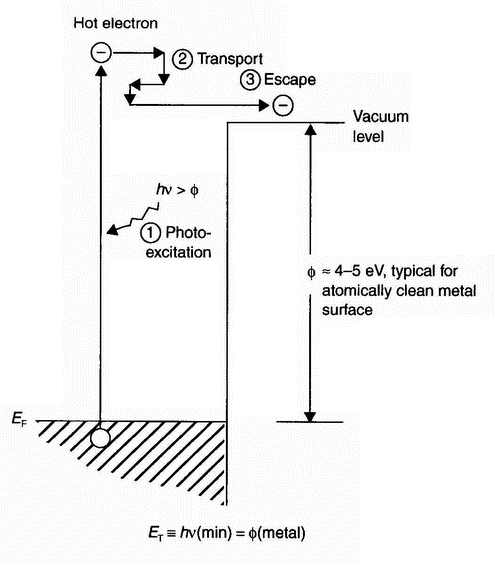 |
Figure 8: Electron energy vs. distance for clean metal surface [15]
Nearly elastic scattering shown for the photo-generated electron within the metal is electron-phonon scattering. IT = minimum photon energy to yield photoemission |
due to the external electric field, while that inside the metal is almost constant (nearly-free-electron model) and increases to the vacuum level at the metal surface. A potential barrier is created at the surface where the solid and external potential energy functions join, whose height and width decrease as the electric field increases, giving higher thermionic emission (Schottky effect). The excess current not associated with thermionic emission arises from quantum mechanical tunnelling of electrons through the potential barrier. More detailed information on field emission can be found elsewhere [15]. The term is exclusively used for the emission of electrons from a solid by tunnelling. Removal of an atom from a surface state by the application of a strong electric field is called field evaporation [16].
Exoemission (EE)
Solid (e.g., metallic) surfaces subjected to mechanical working (e.g. tribological processes, cutting, abrasion) or tensile deformation show emission of electrons that decreases with time [4]. The term 'exoelectron
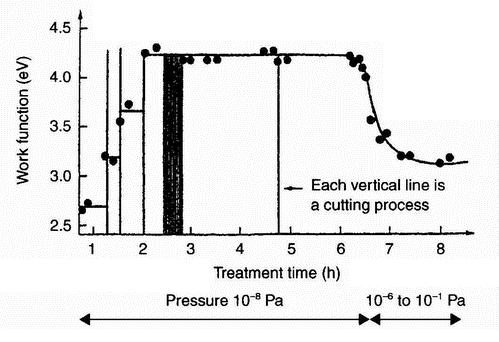 |
Figure 9: Effect of mechanical treatment of an aluminium surface on the alteration of the work function [19] |
emission' (exoemission) originates from investigations into emission from freshly treated metals which was interpreted as being a consequence of exothermal transformation processes of the surface (recrystallisation, phase transformation) [17]. However, according to Fox [18], this effect seems to have been noticed first during the preparation of Geiger counter tubes [19].
The exoelectrons are electrons of low energy spontaneously emitted from most fresh surfaces. EE is used as an inclusive term for emission phenomena that proceed from changes in the work function and from excitation by mechanical energy. For example, an aluminium surface covered with oxide has a work function of about 2.7 eV while the work function of single-crystal aluminium surfaces and evaporated aluminium without oxide coating is approximately 4.3 eV. The influence of mechanical treatment by means of cutting edgeshaped tools on the variation of the work function is represented for aluminium in Figure 9. After appropriate treatment the work function increases to the value measured for the pure uncovered surface and can be maintained constant in an ultrahigh vacuum for several hours. Only by adding small amounts of air does the work function drop to a value that is characteristic for a surface layer covered by oxide. The emission of electrons appears to correlate with these work function changes.
EE appears when a material's surface is disturbed by plastic deformation, abrasion, fatigue cracking, and phase deformation. Emission from freshly formed surfaces reaches a maximum initially,
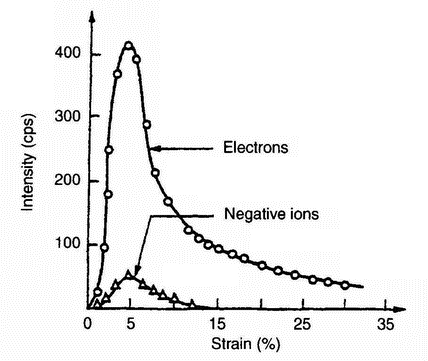 |
Figure 10: Tribostimulated emission rates of electrons and negative ions vs. strain obtained from Al covered with dense aluminium oxide 2000 Å thick: deformation rate = 2.2 x 10 -4s -1 (ref. 22) |
then decays with time [20-22]. It has been observed from both metals and non-metals, and there is strong evidence that oxides or other non-metallic surface layers are necessary for electron emission. It has been demonstrated [23] that the initial stage of EE from abraded aluminium is due to the adsorption of water vapour.
Emission from aluminium covered with a thick oxide film (50-2000 Å thick) was studied by Rosenblum et al. [22] wherein the peak intensity of emission as a function of strain for constant strain rate shifts to a higher strain as the thickness of the oxide decreases. It was also established that the current of negatively charged particles emitted from oxide-covered aluminium consists of both electrons and negative ions (Figure 10) [22]. Some emission of positive ions and photons was detected as well; however, at a strain rate of 2.2 x 10 -4 s -1, the emitted particle flux was weak and almost lost in the background.
The energy ofexoelectrons is typically of the order of 2-3 eV [24]. The mean energy of tribo-stimulated exoelectrons emitted from 2000 Å thick oxide on aluminium is 3.5 eV [22]. The EE rates from nickel specimens are about three times lower than those from aluminium oxide of comparable thickness. Other work [4, 25] indicates that the energy of exoelectrons can be up to 10 eV. However, it is reasonable to assume that the average energy of electrons emitted during rubbing is in the range 1-4 eV.
The mechanism for EE is not well understood, but it seems to be associated with defect formation in a solid and may be augmented by
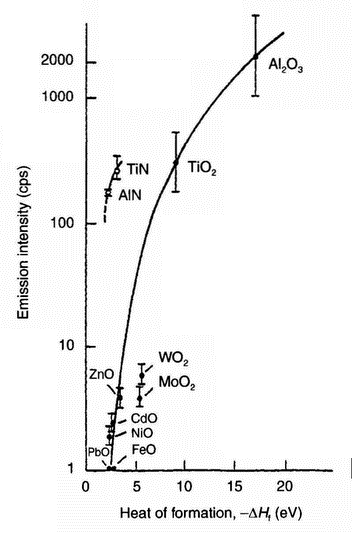 |
Figure 11: Relation between electron emission in oxygen and nitrogen gases and heat of formation of oxides and nitrides of the test metals [30] |
radiation of suitable wavelength [18]. Other work [26] points out that electron emission from metallic surfaces may be attributed to a diffusion-controlled process. In such a process, point imperfections, such as vacancies, in the non-conducting surface layer are considered responsible for the emission. A model of the emission behaviour based on vacancy diffusion [26] predicts emission rates in reasonably good agreement with the experimental data.
EE was also observed from a clean, well-annealed magnesium single crystal in an ultrahigh-vacuum system upon exposure of the surface to both oxygen and chlorine [27]. The emission was found to depend on the flux of oxygen on the surface. The results suggest that the emission is of a chemical origin and not due to surface strain. Interestingly, no emission from aluminium as a result of a flux of oxygen on the surface has been observed, probably because of effects related to sticking coefficient, work function change, and adsorption binding states. For comparison, see also the effect of mechanical treatment of aluminium on the change of work function presented in Figure 9.
Although there are several approaches regarding the source of the energy of exoelectrons, the two most cited are (i) the rearrangement of surface atoms and (ii) surface chemical reactions [28]. The effect of surface chemical reactions has been demonstrated previously [29]. It was found that the emission intensity of exoelectrons decreases as the surrounding gas pressure decreases, suggesting that the electron emission from a fresh metal surface is due to the interaction between the surface and the surrounding active gas species. Further evidence of this came from a study of various metals (Ti, W, Mo, Fe, Al, Ni, Cd, Zn, and Pb) that were cut in oxygen and nitrogen gases at a gas pressure of 3 x 10 -2 Pa and in a vacuum of 4 x 10 -2 Pa [30] (see Figure 11). It was concluded that (i) the emission of electrons from a fresh metal surface in the dark is caused by the interaction of the surface with the surrounding gas and depends on the metal/gas combination; and (ii) electron emission intensity from cut metal surfaces increases with the heat of formation for the reaction of the surface with the surrounding gas.
Two types of electron emission process are considered: (i) triboemission refers to emission of particles during tribological surface damage; (ii) after-emission refers to that from a fresh solid surface after surface damage [30] and depends on the reaction of a gas with the surface. As soon as the mechanical treatment (e.g., cutting, abrasion) stops, triboemission ceases abruptly, since the EE is caused by a high-energy nascent surface. The electron emission from a metal surface is also called 'chemically stimulated exoelectron emission [31] or chemi-emission [32]. Several extensive surveys exist on EE phenomena [20], on exoelectrons [13], on exoelectrons and tribology [14], and on structural and physical changes of solids under mechanical energy influences [4].
An apparent two-step process for emission of electrons from freshly generated surfaces has been reported [33]. Electrons are not ejected from the fresh surface until it is irradiated with photons of insufficient energy to produce photoemission. It was observed in this study that (i) exoelectrons were not observed once illumination ceased and the emission began immediately after the illumination was resumed; (ii) the exoelectron yield after resuming illumination did not depend on the duration of the interruption; and (iii) the yield was essentially identical to that without the interruption. These observations led the authors to suggest that the basic mechanism of EE should be independent of the photoexcitation process. However, other work [34] showed that during the observation of photo-stimulated excitation (PSE) from scratched aluminium, the interruption of photo-stimulation did result in a transient enhancement of the
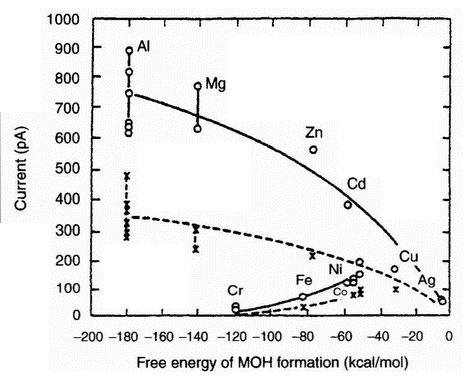 |
Figure 12: Photostimulated emission (PSE) just after abrasion at a relative humidity of 50 ± 10% (o) and at 100 s after abrasion (x), plotted against DFMOH (ref. 36) |
electron yield. Based on this observation, a new model concerning PSE from scratched aluminium was proposed in which there are two competing excitation processes during PSE, the photoexcitation of the electrons at defect-related energy levels above the Fermi level, and the thermal excitation of the lower-lying electrons to the empty defect-related levels. Further research [35] sought to understand how the energy distribution of the emission sources is influenced by scratching and to verify directly the model developed previously [34] by determining the levels of the new sources created. Conventional photoemission from both aluminium and zinc specimens was demonstrated by continuously decreasing the stimulation wavelength from the visible to the ultraviolet region. The yield dependence on the wavelength was found [35] to be considerably different before and after scratching of the specimen surfaces. After scratching, the emission peak was observed at a longer wavelength (lower energy) than the critical wavelength for photoemission from undamaged aluminium or zinc. It was also found that the wavelength of light producing the maximum electron emission was independent of the time after scratching or of the intermission period of the illumination.
In a comprehensive study,36 PSE was measured for over 20 surfaces of metallic materials polished using emery paper in a controlled humidity atmosphere. It was found that, except for silver, the maximum PSE current occurred just after polishing and a monotonic decrease in PSE current followed. The observed decrease in PSE intensity with time was accounted for by the gradual loss of electrons from shallow surface states associated with adsorbed water and oxygen molecules. Interestingly, the PSE intensity from metals of Groups II, III, and IV was relatively high (Figure 12), exhibiting a correlation with the free energy of metal hydroxide formation, while the PSE intensity from transition metals with little free energy for metal hydroxide formation was very low [36].
Electrons and negative and positive ions as well as photons are known to be emitted from oxide-covered, pure, polycrystalline aluminium, nickel, and titanium during tensile deformation. It has been demonstrated that the propagation of cracks in the oxide is uniquely associated with the so-called tribo-stimulated exoemission phenomenon: [37] samples treated in the same fashion yielded very reproducible EE curves, and the EE curve appeared unique for a given oxide-metal alloy system, with potential as an indicator of certain characteristics of the oxide layer.
For metals, it is well established that EE is strongly dependent on the type and pressure of the background gas in which the sample is placed. Evidence for this comes from several sources [28, 38, 39]. Gesell et.al. [29] investigated chemisorptive stimulation of electron emission from fresh magnesium surfaces and concluded that energy given to the excited electrons is almost certainly derived from the reaction of oxygen or water with freshly exposed magnesium surfaces. The observed exoelectron emission currents occur when highly energetic reactions take place on a surface with a low work function. Such surfaces, with work functions of about 1.9 eV, exist at approximate monolayer coverages of oxygen or water on magnesium [37]. These results are consistent with data presented elsewhere [27], where it was found that the EE from a clean, well-annealed magnesium single crystal depends on the flux of oxygen on the surface. Other papers [40, 41] on EE from magnesium, thermally stimulated emission [42-44], and field stimulated emission [45, 46] support the qualitative picture that the energy source for EE is exothermic chemical reactions on the surface. However, the physics is complex, as demonstrated by experiments [42] that found that intense thermally stimulated emission from ZnO required chemisorption of both oxygen and water. Experimental results [47] found a trap level for aluminium 0.65 eV above the Fermi level based on PSE, and tunnelling from that level based on field stimulated emission.
Summary of EE
Exoelectron emission occurs readily from a variety of surfaces and is exceptionally sensitive to the state and history of the surface [46]. This phenomenon has been comprehensively treated in an earlier review [45]. Exoelectrons are particularly important for initiating tribochemical reactions [5, 48-50]. Fatigue wear [47] and wear and migration of solid-film lubricants may be correlated with EE [61]. The mechanisms of EE are very complex and not well understood. Exoemission appears to be connected with the presence of surface films, or possibly the interaction between gas molecules and a metal surface. The hypothesis that chemisorption of oxygen often provides the energy for electron emission seems well justified. It is, however, likely that different combinations of the available mechanical energy, chemical energy, adsorption energy, and thermal energy, balanced against a work function that changes as a result of chemical action and surface combination, operate in different circumstances [13]. When external energy is supplied, in the form of photons, thermal energy, or a static electric field, then the number of ejected electrons increases. Electrons released in this way are stimulated exoelectrons. Chemisorptive stimulation of EE has also been considered. Bearing in mind all the above, the exoelectron emission process can be described as a phenomenon evolving low-energy electrons from mechanically treated solid surfaces; the intensity of EE can be enhanced by heating (TSE), irradiation with ultraviolet light (PSE), and an electric field (FSE). As already mentioned, the deformation and fracture of surfaces emit not only electrons but also other particles (see Figure 2) and photons. Thus fractoemission is an even more complex and challenging process than that of exoelectron emission.
FRACTOEMISSION: Comparison of EE and fractoemission
It has been demonstrated [22] that aluminium covered with a thick oxide layer during tensile deformation emits both electrons and negative ions. The emission rate of electrons as a function of strain is shown in Figure 10. Positive ions and photons are also detected during strain. The surface area created during tensile deformation and fracture of reactive metals (nickel, aluminium, magnesium, titanium, and zirconium) has been determined by the measurement of chemisorptive emission (CSE) associated with oxygen adsorption [52, 53]. The experiments demonstrate the influence of oxide formation on both exoelectron emission and fractoemission.
Tensile deformation of oxide-covered metals is an example of fractoemission generation, which produces positive and negative particles, photons, and phonons (see Figures 4 and 5). Fractoemission can often serve as a sensitive probe of crack growth and may prove a useful tool for investigating molecular and microscopic events accompanying crack formation and for studying the failure modes in a variety of materials [54]. Plastic deformation results in roughening due to intersection of slip lines with the surface. Usually, this causes the break-up of any oxide layer and exposure of fresh micro-surface spots. As a consequence, lower work function surfaces are exposed that yield readily observable increases in photoelectron emission. It has also been shown [63] that chemical stimulated emission measurements
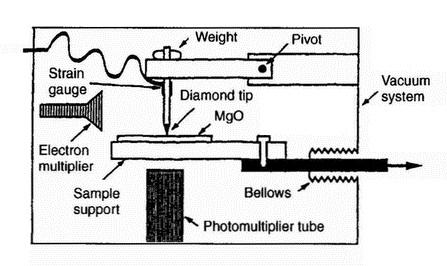 |
Figure 13: Apparatus used for simultaneous photon emission, electron emission, and frictional force measurements in vacuum [55] |
during loading and fracture of aluminium, magnesium, titanium, and zirconium provide information on the increase in fresh surface area. In the case of titanium and zirconium, CSE shows an exponential acceleration in the production of new surface area after the onset of the necking instability, confirmed by surface roughness measurements. For aluminium, CSE and photoelectron emission (PSE) increase in a linear manner during loading, consistent with a nearly constant rate of new surface formation during deformation. In contrast, for magnesium, CSE results indicate that constrained slip also appears to generate an exponential growth in the new surface area with elongation. Interestingly, atomic force microscopy of the naked region in strained titanium samples shows dramatic increases in surface roughness, in near agreement with the CSE results. A model has been developed to interpret these observations.Ba In recent years very extensive and detailed research on fractoemission has been conducted [8, 53-55]. Fractoemission-related work cannot be separated from the exoelectron emission phenomenon and triboemission. A good illustration of this is provided by Figure 13, which shows an apparatus for simultaneous exoelectron emission, photon emission, and fracture measurements, including frictional force.
Brief overview of fractoemission - related research
Fractoemission is defined as the emission of particles and photons during and after fracture of materials. The types of particles that have been observed include electrons, negative and positive ions, as well as neutral species in both ground and excited states plus visible photons. Studies concerning fractoemission54 include the fracture of oxide coating on metals, inorganic crystals and glasses, organic crystals, and adhesive failure (including components). Electrical phenomena / breakdown accompanying fracture have also been studied.
Fractoemission associated with the fracture of oxides and consequent emission-related phenomena has been widely investigated. The initial emission of particles is due to the adsorption of water vapour and the subsequent decay in emission is associated with oxidation [23] of fresh metal surfaces. The close correlation between the emission of particles and oxidation of the surface suggests that kinetic studies of the early stages of oxidation might fruitfully be pursued using fractoemission.
Extensive research [56-59] has studied electron emission from anodically oxidised aluminium subjected to tensile deformation in a vacuum. The major emphasis of that work was PSE and dark emission. It was concluded that PSE from aluminium deformed in tension resulted from the reduction of the work function due to the adsorption of oxygen and water molecules on the fresh surface exposed by slip plane dislocations terminating at the surface. A large increase in current was observed when the sample fractured due to the exposure of fresh metal on the fracture faces. PSE plays a similar role in both fractoemission and triboemission [60].
A significant study [61] of several stimulated exoelectron emission processes from oxide-covered metals came to the following conclusions regarding fractoemission:
- emission begins at a strain of less than 0.2% when the strain rate is 2.2 x 10 -4 s -1
- emission is observed only at finite or non-zero strain rates, and ceases abruptly once deformation is stopped
- emission occurs discontinuously, i.e., in bursts
- during continuous deformation in total darkness at a constant strain rate, the electron emission rate increases rapidly to a maximum, then decreases monotonically
- even under the most favourable conditions, the emission yield of electrons is extremely low.
Dickinson [54] reviewed and discussed in detail features concerning fractoemission from adhesive failure. Another review [55] describes two novel, dynamic probes of fracture, wear, and deformation during tribological loading, that provide insight into energy dissipation mechanisms associated with fracture, flow, and adhesive detachment. The use of these probes is demonstrated during abrasive wear on single-crystal MgO by diamond and tungsten carbide.
Summary of fractoemission
As major studies concerning fractoemission include fracture of oxide coatings on metals and fracture of crystals, it is feasible to state that
Table 3. Summary of fractoemission references |
Ref. | Material system and atmosphere | Stress type, strain rate | Measurement system | Emission level |
61 | Oxide-covered aluminium, nickel, and titanium. Vacuum ~10 -6 Pa | Tensile, 0.5 to 10 -4 s -1 | Single-event channel electron multiplier and energy-distribution spectrometer | Up to 450 counts/s |
62 | 'Commercial' and 'high- purity' aluminium. Mixed atmosphere of 1 % methane and 99% He | Tensile, 0.063 to 0.159 in/min | Geiger-Muller flow counter | Up to 200 counts/s |
37, 63 | Bare and oxide- covered 2024 Al alloy. 10sup> -6 Torr vacuum | Tensile, until rupture, 0.014% s -1 | Single-event channel electron multiplier | Up to 1100 counts/s |
8 | Bare and oxide- covered 2024 Al alloy. Vacuum <10 -7 Pa | Tensile, constant strain rate | Bayer-Alpert gauge for total gas pressure. Quadrupole spectrometer for gas partial pressure | Up to 1.6 x 10 -7 Torr total pressure increase |
64 | Anodised 1350 Al alloy. 10 -4 Pa vacuum | Tensile, 0.014-25% s -1 | Single-event channel electron multiplier | Up to 50 counts/s |
65 | Anodised 1350 Al alloy 10 -4 Pa vacuum | Tensile, 0.2% s -1 | Single-event channel electron multiplier to count 'per acoustic- emission burst' | Up to 8 counts 'per burst' |
66, 67 | Alumina-filled epoxy, glass-bead-filled polybutadiene, sucrose, SiO2, MgF2, 10 -5Pa vacuum | Tensile and three-point bending until fracture | Single-event channel electron multiplier | Up to 14,000 counts/s for sucrose |
68 | LiF and CaF2. Up to 400°C in air | 2 min indentation (98-294 N) | Electro-conductive indenter tip with ±15 V bias | Arbitrary units |
69 | LiF and NaF2 | Three-point bending until fracture, 70 µm/s | 20 V floated ion collector feeding an electrometer | Up to 170nA |
70, 71, 72 | Stainless steel rod embedded in epoxy. 10 -5 Pa vacuum | Core-rod pullout, 2 mm/min | Electro-optic channel electron multiplier | Up to 2000 counts/s |
73 | High-density polyethylene | Tensile, 30% s -1 | Two channel electron multipliers | Up to 1100 counts/s |
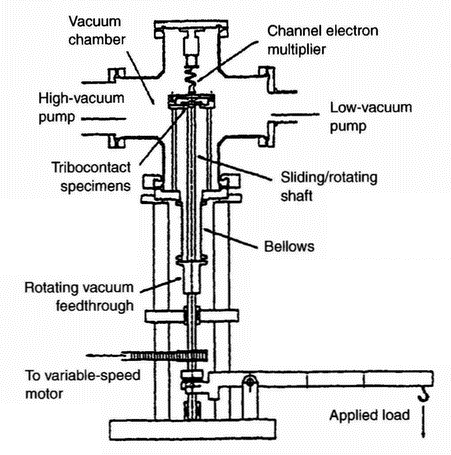 |
Figure 14: Features of triboemission instrument [90] |
fractoemission forms a bridge between metal and ceramic triboemission. Table 3 summarises a variety of experimental set-ups used by researchers examining the fractoemission process. The research reviewed in Table 3 shows approaches to measuring fractoemitted electrons, negative and positive ions, neutral particles, photons, and acoustic emission. Although the usual mechanical excitation is tensile strain of the material, interphase debonding and indentation are also sources of fractoemission. In general, particle measurement systems are based on channel electron multipliers in the pulse-counting mode which operate in medium to high vacuum. The various fractoemission features, summarised in Table 3, demonstrate that alkali metal halides [66-69], selected polymers [70-73], and some ceramic- and polymer-matrix composites [66] show fractoemission. In the case of metals a surface oxide layer is required for fractoemission as exemplified by the widely studied metal aluminium [7, 8, 26, 37, 61, 63-65]. There is also a correlation between the thickness of the oxide layers and the fractoemission process, and the relationship between crack propagation, detected by acoustic emission, and fractoemission has been demonstrated.
RESEARCH APPARATUS AND TRIBOEMISSION
One of the most important issues concerning triboemission is the specific apparatus (an example is shown in Figure 14) and measurement techniques. Table 4 summarises the relevant information along with research results and presents a wide variety of experimental set-ups used in the research reviewed here.
Conditions of severe wear were employed for most of these experiments, e.g., scratching a rotating disc with a diamond stylus [74-83, 87]. Variables that may relate to wear processes, including sliding speed [74, 76], normal load [75], material hardness [76], and the environment of hydrocarbons under boundary lubrication conditions [79, 80], are correlated with charge intensities. For example, triboemission of both negatively and positively charged particles was measured during the scratching of various metals, ceramics, and polymers under boundary lubrication conditions with saturated hydrocarbons [80]. In general, the coefficient of friction does not correlate with the emission level [76]. However, surface damage is an important factor for triboemission, but measurements often are not reported [83] or correlated with the recorded triboemission level.
CONCLUSIONS
Based on previous work [37, 55] the general characteristics of the emission accompanying cleavage or fracture are as follows:
- emission begins and is most intense during fracture; this means that the rate of production and decay of excitations is highest
during crack propagation
- the vast majority of the detected negative charge consists of electrons
- fractoemission continues after fracture, indicating that the fracture surfaces are in an activated state which relaxes, at least in
part, via non-radiative processes.
The creation of fresh surfaces under dry and/or boundary conditions of solids results in the emission of electrons, negative and positive ions, neutral particles, photons, gases, and even X-rays. The term 'triboemission' is applied to all these processes. To understand better the complexity of these processes as well as their significance for boundary lubrication, the present paper has reviewed not only works directly encompassing triboemission issues but has also included selected information on related phenomena.
The diversity of experimental conditions makes it difficult to compare different results. Based primarily on the work of Nakayama and Hashimoto [75-77, 81-83] the following conclusions can be drawn:
- Triboemission of both negatively and positively charged particles appears during scratching of metals, ceramics, and polymers under boundary lubrication conditions with and without saturated hydrocarbons. More negatively charged particles are emitted than positively charged ones. The emission characteristics depend on the insulating capacity of the solid and the emission intensity increases in the order: conductor < semiconductor < insulator.
- The emission occurs in bursts and ceases when scratching stops. Emission can also continue after abrasion stops. The emission intensity of both negatively and positively charged particles in insulators decreases as the hardness increases. For metals, the increasing emission intensity correlates with the thickness of oxide films on the metal. The charge ratio of negative to positive particles under boundary lubrication exceeds the value of 2 in saturated hydrocarbons (except in polytetrafluoroethylene).
- Triboemission from ceramic materials depends on gas species and pressure. Emission shifts from minimum to maximum when the gas pressure is varied in the range 10-104 Pa.
- Surface contacts between solids under rubbing conditions give rise to specific physical effects, which, in combination with the tribological system environment, provide important information for understanding the extremely complex and detailed issues of the boundary lubrication process.
- More sophisticated work is needed to account for the interesting and sometimes surprising results obtained in typical experiments under boundary lubrication conditions.
Table 4. Triboemission apparatus: characteristic features and results from relevant research on the triboemission process |
Ref. | Material system and atmosphere† | Normal force*(N) | Speed * (cm/s) | Maximum charged particle emission level† | Detection system† |
23 | Stainless steel brush abrading high purity Al. Air, O2, N2, and atmospheres at 10 -8 to 10 -5 Torr | (NR) | (NR) | 6.5 x 10 -16 A for 10 -5 Torr vacuum | (a) |
74 | Diamond stylus scratching aluminium oxide film. Ambient atmosphere | 1.0 | 5.5 | Arbitrary units | (b) |
75 | Diamond stylus scratching metals and ceramics. Ambient atmosphere | 0.5 | 6.3 | Arbitrary units | (b) |
76 | Diamond stylus scratching various metals and ceramics, glass, AI2O3 films, polymers, and mica. Various atmospheres | 0.5-1.5 | 7-12 | Arbitrary units | (b) and (c) |
77 | Diamond stylus scratching ceramics. Ar, He, O2, N2, and dry air atmospheres at 10 -5 Pa | 1.5 | 3 | Arbitrary units | (b) and (c) |
78 | Stainless steel pin on graphite disc followed by UV irradiation. 10 -8 - vacuum | 7.9 | 0.6 | 4x 10 -15 A | (d) |
79, 80 | Diamond stylus scratching metals, ceramics, polymers, and semiconductors. Submerged in hydrocarbon liquids | 1 | 5.3 | 70 pC/s for negative emission (1 s), (h), (i) | (b) |
81 | Diamond stylus scratching Al2O3, Si3N4, and ZrO2; Hydrocarbon gas atmospheres at 105 Pa | 1.5 | 3 | 350 pC/s for negative and 160 pC/s for positive emissions (6 ms) (h), (i) | (b) and (c) |
82 | Diamond stylus scratching Al2O3, Si3N4, and ZrO2. He, Ar, and 02 atmosphere at 10 -2 to 10 -3 Pa | 1.5 | 6 | 1150 pC/s for negative and 950 pC/s for positive emissions (1 s) (h), (i) | (b) and (c) |
83 | Diamond stylus scratching Al2O3 and Si3N4 (g) n-Butane gas atmospheres at 1 to 105 Pa | 1.5 | 6 | 600 pC/s for negative and 500 pC/s for positive emissions (h) | (b) and (c) |
84, 85 | Alumina ball sliding on amorphous and hydrogenated amorphous carbon films. Dry air atmosphere at 5 mPa | 0.44 | 2.2 | 500 counts/s for negative emissions | (e) |
86 | Alumina ball sliding on amorphous and hydrogenated amorphous carbon films. Dry air atmosphere at 5 mPa | 0.2 | 5.2 | 160 pC for negative emissions (1 s) (i) | (b), (c) and (f) |
87 | Diamond stylus on MgO disc. Vacuum below 10 -4 Pa | 0.1 | 0.25 | 4 x 105 counts/s | (e) |
88 | Diamond stylus on reciprocating MgO, sapphire, SiO2, and a-quartz. Air, N2, and O2 flow at 1.4 atm | 30-100 | (NR) | 24 pA negative emission as collected from gas flow | (j) |
*(NR) = not reported.
†(a) Be-Cu particle multiplier feeding an electrometer;
(b) electrode on diamond stylus at 0.5 mm from scratched surface, ±15 V biasing with respect to surface;
(c) simultaneous photon measurement by photomultiplier tube;
(d) microchannel plate feeding an electronic picoammeter;
(e) secondary electron multiplier feeding a rate counter;
(f) electrostatic voltmeter for surface potential measurement;
(g) 4 mm alumina ball sliding on alumina and Si3N4, for photon emission only (6 N load);
(h) emission for scratched alumina;
(i) as accumulated charges for reported period;
(j) Cu collector feeding floated electrometer.
|
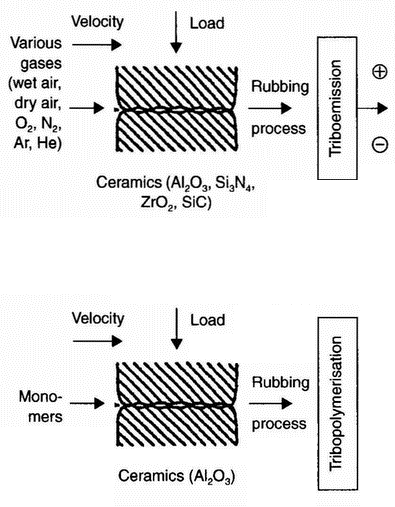 | Known
Positively charged particles
Negatively charged particles
- Selected polycondensation and vinyl-type monomers reduce wear significantly
- OctadecyI vinyl ether, which polymerises according to cationic mechanism, does not reduce wear of Al2O3
|
Unknown
- Types of species
- Their energy
- Proportion of electrons to other negative particles
- Energy of electrons and other negative particles
- Role of negatively charged particles in initiation of anionic tribopolymerisation
- Role of positively charged particles in initiation of
- polycondensation and
- cationic vinyl tribopolymerisation
Figure 15: Overview of tribopolymerisation research | |
The possibility that chemical reactions in solids can be initiated by mechanical deformation and/or friction has been considered for over a century. The historical research related to both mechanochemistry and tribochemistry has been reviewed previously [4, 18]. The mechanical initiation of chemical reactions is of particular importance in the boundary lubrication process of both metals and ceramics.
More detailed information concerning this issue with particular emphasis on tribopolymerisation as a new boundary lubrication mechanism can be found in the literature [50, 89-97]. This general rationale and approach is very interesting from the viewpoint of a monomer substrate interaction and is both complex and very effective in tribological wear reduction. Figure 15 depicts diagrammatically the known and unknown factors that relate to the complexity of the triboemission/tribopolymerisation issue. The authors' most recent research presents experimental work seeking a better understanding of triboemission and its role in tribochemistry [90], including a new instrument to measure intensity and retarded-energy spectra of positively and negatively charged triboemitted particles. Experimental results are reported on triboemission intensity from the sliding contact of diamond on three related materials: alumina, single-crystal sapphire (Al2O3), and aluminium. These results are discussed with a focus on the possible role of charged particle emission in tribochemical processes and, in particular, in tribopolymerisation as an effective mechanism of ceramic lubrication. Another work98 aims at making connections between triboemitted particles in ceramic systems and tribochemical reactions, in particular tribopolymerisation. It compares the tribochemical behaviour of model monomers in diamond-on-alumina and alumina-on-alumina systems under different friction conditions. The results show a very high reactivity of the monomers studied even under very mild rubbing conditions. Extremely high reactivity of the compounds when applied as pure lubricants led to the increased wear rate of alumina. However, monomers applied as 1% solutions showed very effective antiwear and antifriction properties.
A review has been published [99] of the practical applications of tribopolymerisation, a novel concept of molecular design. Possible applications include: ashless antiwear or 'lubricity' additives for fuels, including gasoline, diesel, and jet fuel; the development of ashless lubricants for existing and future automotive engines to reduce exhaust catalyst poisoning and environmental emissions; the lubrication of ceramic engines (e.g., low-heat-rejection diesel engines) or ceramic components; machining and cutting applications using thin films to reduce friction and ceramic tool wear; special 'minimalist' pre-treatment compositions for assembling and running in of engines; vapour-phase applications of this technology to high-temperature gaseous systems or to fuel injector wear problems associated with the use of natural gas engines; and an enabling technology in the development of new engines and propulsion systems.
Acknowledgment
Authors express their appreciation to the Surface Engineering and Tribology Program of the US National Science Foundation for its support of this work under project number CMS-953 1661.
References
- Suh, N.P. Tribophysics, Prentice-Hall, Englewood Cliffs, NJ, 1986.
- Kajdas, C., 'Physics and chemistry of tribological wear', Proc. 10th Int. Coll. Tribology, 9-11 January 1996, Technische Akademie Esslingen, Östfildern.
- Moore, M.A., 'Energy dissipation in abrasive wear', in Wear of Materials, ASME, 1979, pp. 636-8.
- Heinicke, G., Tribochemistry, Carl Hanser Verlag, Munich and Vienna, 1984.
- Kajdas, C., 'Importance of anionic reactive intermediates for lubricant component reactions with friction surfaces', Lubrication Science, 6 (1994) 203-28.
- Nakayama, K., Triboemission from wearing solid surfaces', ITC Proceedings, Yokohama 1995, Satellite Forum on Tribochemistry, 28 October 1995, Tokyo.
- Dickinson, J.T., Snyder, D.B., and Donaldson, E.E., 'Acoustic emission and electron emission during deformation of anodized aluminum', J. Vac. Sci. Technol., 17 (1980) 429-32.
- Larson, LA., Dickinson, J.T, Braunlich, P.P., and Snyder, D.B., 'Emission of neutral particles from anodized aluminum surfaces during tensile deformation', J. Vac. Sci. Technol., 16 (1979) 590-3.
- Glaeser, W.A., Material for Tribology, Tribology Series 20, Elsevier, Amsterdam, 1992.
- Buckley, D.E., Surface Effects in Adhesion, Friction, Wear and Lubrication, Tribology Series 5, Elsevier, Amsterdam, 1981.
- Bube, R.H., 'Electrons in solids', Encyclopedia of Physical Science and Technology, Elsevier, Amsterdam, 1992, vol. 5, pp. 737-60.
- Cardona, M., and Ley, L. (ed.), Photoemission in Solids I, Topics in Applied Physics Series 26, Springer-Verlag, Berlin, 1978.
- Rabinowicz, E., 'Exoelectrons', Sci. Amer., 236 (1977) 74-81.
- Ohmae, N., Nakayama, K., and Mori, S., 'Exoelectrons and tribology', Jpn. J. Trib., 40 (1995) 355-62.
- Escher, J.S., 'NEA semiconductor photoemitters', Semiconductors and Semimetals, ed. R.K.Willardson and A.C. Beer, Academic Press, New York, 1981, Vol. 15, Chap. 3, pp. 195-300.
- Bell, A.E., and Barofski, D.R, 'Field emission and field ionization', Encyclopedia of Applied Physics, CH Publishers, New York, 1993, Vol.6, pp. 379-91.
- Kramer, J., Der metallische Zustand, Vanderhoek und Reprecht, Goettingen, 1950.
- Fox, P.G., 'Review of mechanically initiated chemical reactions in solids', J. Mater. Sci., 10 (1975) 340-60.
- Born, D., Dissertation, Univ. Berlin, 1975.
- Grunberg, L., 'A survey of exoelectron emission phenomena', Appl. Br. J. Phys., 9 (1958) 85-93.
- Verman, C., 'Registration and mapping of plastically deformed metal surfaces by means of photoelectrons', Mater. Sci. Eng., 4 (1969) 329-42.
- Rosenblum, B., Braunlich, P., and Himmel, L., 'Spontaneous emission of charged particles and photons during tensile deformation of oxide-covered metals under ultra-high conditions', J. Appl. Phys., 48 (1977) 5262-73.
- Ramsey, J.A., 'The emission of electrons from aluminum abraded in atmospheres of air, oxygen, nitrogen and water vapour', Surf. Sci., 8 (1967) 313-22.
- Chaikin, S.W., 'On frictional polymer', Wear, 10 (1967) 49-60.
- Kobzev, N.I., Exoelectronic Emission, The Foreign Literature, Moscow, 1962.
- VonVoss,W.D.,and Brotzen, F.R.,'Electron emission from plastically strained aluminum', J. Appl. Phys., 30 (1959) 1639-45.
- Ferrante, J., 'Exoelectron emission from a clean, annealed magnesium single crystal during oxygen adsorption', ASLE Trans., 20 (1977) 328-32.
- Connelly, M., and Rabinowicz, E., 'Detecting wear and migration of solid-film lubricants using simultaneous exoelectron emission', ASLE Trans., 26 (1983) 139-43.
- Gesell, T.F, Arakawa, E.T., and Callcott, T.A., 'Exoelectron emission during oxygen and water chemisorption on fresh magnesium surface', Surf. Sci., 20 (1979) 174-8.
- Nakayama, K., Leiva, J.A., and Enomoto, Y., 'Chemi-emission of electrons from metal surfaces in the cutting process due to metal/glass interactions', Trib. Int., 28 (1995) 505-15.
- Sato, N., and Seo, M., 'Chemically stimulated exo-emission from a silver catalyst', Nature, 216 (1967) 361-2.
- Moucharatieh, N., and Olmsted, J., 'Chemiemission from cesium-oxygen surface reaction', J. Phys. Chem., 75 (1971) 1928-36.
- Arnott, D.R., and Ramsey, Y.A., 'Electron emission from anodically oxidized aluminum due to tensile deformation', Surf. Sci., 28 (1971) 1-18.
- Shigekawa, H., and Hyodo, S., 'Storage effect in photostimulated exoelectron emission from scratched aluminum', Jpn. J. Appl. Phys., 21 (1982) 1278-82.
- Shigekawa, H., Iwatsu, R., Okada, M., and Hyodo, S., 'Change of energy level distribution of photoemission sources in Al and Zn caused by scratching', Jpn. J. Appl. Phys., 22 (1983) 42-5.
- Enomoto, Y., and Hashimoto, H., 'Photo-stimulated exoelectron from abraded metals in atmospheric conditions', Jpn. J. Tribol., 35 (1990) 381-9.
- Dickinson, J.T, Braunlich, P.P., and Larson, LA., 'Characteristic emission of negatively charged particles during tensile deformation of oxide-covered aluminum alloys', Appl. Surf. Sci., 1 (1978) 515-37.
- Ramsey, J.A., 'Exoelectron emission from abraded metal surfaces at high and ultrahigh vacuums', J. Appl. Phys., 37 (1966) 452-4.
- Hoening, S.A., and Pope, R.A., 'Emission of high-energy electrons during alloy evaporation process on hot metal filaments', Appl. Phys. Lett., 14 (1969) 271-2.
- Dus-Sitek, M., Olszowski, Z., and Sujak, B., 'Optically stimulated exoelectron emission for determining the degree of hydrogen embrittlement of nickel', Jpn. J. Appl. Phys., 24 (1985) 176-86.
- Markowska, L., and Sujak, B., 'Possibilities of optically stimulated exoemission registration in the nano- and microsecond range from CsCI', Phys. Stat. So/.,121 (1991)273-8.
- Kreigseis, W., and Sharman, A., 'Exoelectron emission of ZnO', Phys. Stat. Sol. (a), 29 (1975) 407-14.
- Tomita, A., Fukuda, Y., Kutomi, Y, and Takeuchi, N., 'Emission processes in thermally stimulated exoelectron emission and thermoluminescence of KCI-Ag single crystals', Phys. Stat. Sol. (b), 158 (1990) 383-98.
- Kamada, M., Yoshiara, K., and Tsunumi, K., 'Energy spectra of thermally stimulated exoelectrons from KCI:TI irradiated at 77 K', Jpn. J. Appl. Phys., 23 (1984)286-90.
- Robertson, A.J.B., 'Exoelectron emission', Int. J. Electronics, 51 (1981) 607-19.
- Hibbert, D.B., and Goodman, A., 'Theoretical study of field-stimulated exoelectron emission', Int. J. Electronics, 59 (1985) 701-9.
- March, P.A., and Rabinowicz, E., 'Exoelectron emission for the study of surface fatigue wear', ASLE Trans., 20 (1977) 315-20.
- Kajdas, C., 'About a negative-ion concept of the antiwear and antiseizure action of hydrocarbons during friction', Wear, 101 (1985) 1-12.
- Kajdas, C., 'About an anionic-radical concept of the lubrication mechanism of alcohols', Wear, 116 (1987) 167-80.
- Furey, M.J., Kajdas, C., Kempinski, R., and Tripathy, B., 'Action mechanism of selected vinyl monomers under boundary lubrication of an alumina-on-alumina system', Lubrication Science, 10 (1997) 3-25.
- Connelly, M., and Rabinowicz, E., 'Detecting wear and migration of solid-film lubricants using simultaneous exoelectron emission', ASLE Trans., 26 (1983) 139-43.
- Sujak, B., and Gieroszynski, A., 'Exoelectron emission of surface-oxidized nickel during plastic deformation in vacuum', Acta Phys. Polon., A37, 5 (1970) 733-40.
- Dickinson, J.T, Jensen, L.C., Langford, S.C., and Hoagland, R.G., 'Chemisorptive electron emission as a probe of plastic deformation in reactive metals', J. Mater. Res., 9 (1994) 1156-65.
- Dickinson, J.T., 'Fracto-emission from adhesive failure', Adhesive Bonding, ed. L.M. Lee, Plenum Press, New York, 1991, pp. 395-423.
- Dickinson, J.T., Scudiero, L, Yasuda, K., Kim, M.-W., and Langford, S.C., 'Dynamical tribological probes: particle emission and transient electrical measurements', Trib. Lett., 3 (1997) 53-67.
- Sujak, B., Gieroszynski, A., and Pega, F, 'Effect of oxide barrier layer and measuring parameters on the initial deformation e0 yielding photostimulated exoelectron emission in vacuum from plastically deformed aluminum', Acta Phys., Polon., 28 (1965) 61-71.
- Gieroszynski, A., and Sujak, B., 'Exoelectron emission in vacuum in the absence of light during plastic deformation of aluminum thickly coated with oxide', Acta Phys. Polon., 28 (1965) 311-27.
- Sujak, B., Gieroszynski, A., and Gajda, R., 'Effect of oxide and of measuring parameters on the decay of photostimulated (exo)electron emission in vacuum from plastically deformed aluminum (Part I)', Acta Phys. Polon., 28 (1965) 329-35.
- Gieroszynski, A., and Sujak, B., 'Effect of oxide and measuring parameters on the decay of photostimulated (exo)electron emission in vacuum from plastically deformed aluminum (Part II)', Acta Phys. Polon., 28 (1965) 337-42.
- Arnott, D.R., and Ramsey, J.A., 'Electron emission from anodically oxidized aluminum due to tensile deformation', Surf. Sci., 28 (1971) 1-18.
- Rosenblum, B., Carrico, J.P., Braunlich, P., and Himmel, L., 'Measurement of triboemission from oxide-covered metals', J. Phys. E, 10 (1977) 1056-62.
- VonVoss,W.D.,and Brotzen, F.R.,'Electron emission from plastically strained aluminum', J. Appl. Phys., 30 (1959) 1639-45.
- Doering, D.L., Oda, T, Dickinson, J.T., and Braunlich, P.F, 'Characterization of anodic oxide coatings on aluminum by tribostimulated exoemission', Appl. Surf. Sci.,3 (1979) 196-210.
- Dickinson, J.T., Donaldson, E.E., and Snyder, D.B., 'Acoustic emission and electron emission during deformation of anodized aluminium', J. Vac. Sci. Technol., 17, 1 (1980) 429-32.
- Dickinson, J.T, Donaldson, E.E., and Snyder, D.B., 'Emission of electrons and positive ions upon fracture of oxide films', J. Vac. Sci. Technol., 18, 2 (1981) 238-42.
- Dickinson, J.T, Jensen, L.C., and Jahan-Latibari, A., 'Fracto-emission: the role of charge separation', J. Vac. Sci. Technol., A2, 2 (1984) 1112-16.
- Yoo, K.C., Rosemeier, R.G., Dickinson, J.T, and Langford, S.C., 'Anisotropic effects on fractoemission from MgF2 single crystals', Appl. Phys. Lett., 55, 4 (1989) 354-6.
- Enomoto, Y., and Hashimoto, H., 'Emission of electrons and ions during indentation and scratching of brittle crystals at elevated temperatures up to 400°C in air', Proc. Jpn. Int. Trib. Conf., Nagoya, 1990, pp. 1189-94.
- Dickinson, J.T, Jensen, L.C., Langford, S.C., and Hirth, J.P., 'Atomic and molecular emission following fracture of alkali halides: a dislocation driven process', J. Mater. Res., 6, 1 (1991) 112-25.
- Ma, Z., and Dickinson, J.T, 'Fracto-emission from embedded surfaces', J. Appl. Phys., 70, 9 (1991) 4797-807.
- Zimmerman, K.A., Langford, S.C., and Dickinson, J.T, 'Electrical transients during interfacial debonding and pullout of a metal rod from an epoxy matrix', J. Appl. Phys., 70, 9 (1991) 4808-15.
- Dickinson, J.T, Jensen, L.C., Lee, S., Scudiero, L., Langford, S.C., 'Fractoemission and electrical transients due to interiacial failure', J. Adhesion Sci. Tech., 8, 11 (1994) 1285-309.
- Dickinson, J.T., and Jensen, L.C., 'Fractoemission from high density polyethylene: bond breaking versus tribological stimulation', J. Appl. Phys., 73, 6 (1993)3047-53.
- Nakayama, K., Hashimoto, H., and Fukuda, Y., Triboemission from aluminum oxide film in atmosphere', Proc. Jpn. Int. Trib. Conf., Nagoya, 1990, pp. 1141-6.
- Nakayama, K., and Hashimoto, H., 'Triboemission from various materials in atmosphere', Wear, 147 (1991) 335-43.
- Nakayama, K., Hashimoto, H., and Susuki, T, Triboemission of charged particles and photons from solid surfaces during frictional damage', J. Phys. D, 25 (1992)303-8.
- Nakayama, K., and Hashimoto, H., Triboemission of charged particles and photons from wearing ceramic surfaces in various gases', Trib. Trans., 35, 4 (1992) 643-50.
- Tagawa, M., Mori, M., Ohmae, N., Umeno, M., and Takenobu, S., Tribe- and photo-stimulated electron emission from graphite', Trib. Int., 26, 1 (1993) 57-60.
- Nakayama, K., Triboemission of charged particles from various solids under boundary lubrication conditions', Wear, 178 (1994) 61-7.
- Nakayama, K., and Hashimoto, H., Triboemission of electrons and ions during wear of solids under boundary lubrication with saturated hydrocarbon liquids', Trib. Trans., 38, 3 (1995) 541-8.
- Nakayama, K., and Hashimoto, H., Triboemission of charged particles and photons from wearing ceramic surfaces in various hydrocarbon gases', Wear, 185, 4(1995)183-8.
- Nakayama, K., and Hashimoto, H., 'Effect of surrounding gas pressure on triboemission of charged particles and photons from wearing ceramics surfaces', Trib. Trans., 38, 1 (1995) 35-42.
- Nakayama, K., and Hashimoto, H., Triboemission, tribochemical reactions, and friction and wear in ceramics under various n-butane gas pressures', Trib. Int., 29, 5 (1995) 507-15.
- Nakayama, K., and Ikeda, H., Triboemission characteristic of electrons during wear of amorphous carbon and hydrogenated amorphous carbon films in a dry air atmosphere', Wear, 198 (1996) 71-6.
- Nakayama, K., and Hashimoto, H., 'Friction, wear, and triboelectron emission of hydrogenated amorphous carbon films', Trib. Trans., 40, 3 (1997) 507-13.
- Nakayama, K., Bou-Said, B., and Ikeda, H., Tribo-electromagnetic phenomena of hydrogenated carbon films - tribo-electrons, -ions, -photons, and -charging', J. Trib., 119 (1997) 764-8.
- Kim, M., Langford, S.C., and Dickinson, J.T., 'Electron and photon emission accompanying the abrasion of MgO with diamond', Trib. Lett., 1 (1995) 147-57.
- Scudiero, L., Dickinson, J.T., and Enomoto, Y., The electrification of flowing gases by mechanical abrasion of mineral surfaces', Phys. Chem. Minerals, 25 (1998) 566-73.
- Furey M.J., Ritter, A.L., Vick, B., Molina, G.J., and Kajdas, C., Triboemission and surface temperatures generated in a ball-in-cone tribocontact', Proc. 5th Int. Tribology Conference, Austrib '98, ed. D.J. Hargreaves and W. Scott, Institution of Engineers, Australia, 1998, pp. 257-62.
- Molina, G., Furey, M.J., Ritter, A., and Kajdas, C., Triboemission from alumina, single crystal sapphire, and aluminum', Proc. Nordtrib 2000, vol. 2, pp. 561-70, VTTTech. Res. Centre of Finland.
- Furey, M.J., and Kajdas, C., Tribopolymerization as a lubrication mechanism for high-energetic contacts of solids', Proc. 6th Int. Tribology Colloq., 12-14 January 1988, Ostfildern, Germany
- Furey, M.J., and Kajdas, C., 'Models of tribopolymerization as an anti-wear mechanism', Proc. Jpn. Int. Trib. Conf., Nagoya, 1990, pp. 1089-94.
- Furey, M.J., and Kajdas, C., 'Tribopolymerization as a novel approach to ceramic lubrication', Proc. 4th Int. Symp. on Ceramic Materials and Components for Engines, 1991, pp. 1211-18.
- Tripathy, B.S., Furey, M.J., and Kajdas, C., 'Mechanism of wear reduction of alumina by tribopolymerization', Wear, 181-183 (1995) 138-47.
- Kajdas, C., Laflech, P., Furey, M.J., Hellgeth, J.W., and Ward, T.C., 'A study of tribopolymerization under fretting contact conditions', Lubrication Science, 6 (1993) 51-89.
- Furey, M.J., Ghasemi, H., Tripathy, B.S., Kajdas, C., Kempinski, R., and Hellgeth, J.W., Tribochemistry of the antiwear action of a dimer acid/glycol monoester on alumina', Trib. Trans., 37 (1994) 67-74.
- Kajdas, C., Furey, M.J., and Kempinski, R., 'Tribopolymerization: II. NIRAM approach to the antiwear action of addition-type monomers', 2nd Int. Symp. Tribochemisfry, 15-17 September 1997, Janowice, Poland.
- Kempinski, R., Malosse, P., Furey, M.J., Kajdas, C., Molina, G.J., and Tripathy, B.S., 'Effect of triboemitted particles on the tribological behavior of selected monomers', Proc. Nordtrib 2000, vol. 3, pp. 867-76, VTT Tech. Res. Centre of Finland.
- Furey, M.J., Kajdas, C., and Kempinski, R., 'Applications of the concept of tribopolymerization in fuels, lubricants, metalworking, and "minimalist" lubrication', Proc. Nordtrib 2000, vol. 3, pp. 583-92, VTT Tech. Res. Centre of Finland. This paper was first presented at the 12th International Tribology Colloquium, Technische Akademie Esslingen, Germany.
|